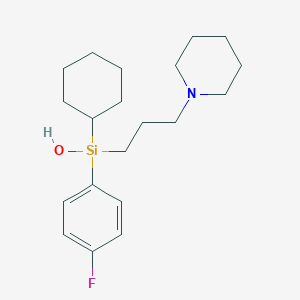
pFHHSiD
Overview
Description
The compound para-fluorohexahydrosiladifenidol, commonly referred to as p-F-HHSiD, is a synthetic chemical compound with the molecular formula C20H32FNOSi. It is known for its selective antagonistic properties on muscarinic receptors, particularly the M3 subtype .
Scientific Research Applications
Para-fluorohexahydrosiladifenidol has several scientific research applications:
Chemistry: It is used as a selective antagonist in studies involving muscarinic receptors.
Biology: The compound is employed in research on cellular signaling pathways and receptor interactions.
Medicine: It has potential therapeutic applications in the treatment of diseases involving muscarinic receptors.
Industry: The compound is used in the development of pharmaceuticals and other chemical products.
Mechanism of Action
Target of Action
pFHHSiD, also known as para-Fluoro-hexahydrosila-difenidol, primarily targets muscarinic receptors , specifically the M3 subtype . Muscarinic receptors are a type of G protein-coupled receptor that plays a crucial role in various physiological functions, including neural and non-neural signal transmission .
Mode of Action
This compound acts as an antagonist at the M3 muscarinic receptors .
Preparation Methods
Synthetic Routes and Reaction Conditions
The synthesis of para-fluorohexahydrosiladifenidol involves the reaction of cyclohexyl (4-fluorophenyl) [3-(1-piperidinyl)propyl]silanol with appropriate reagents under controlled conditions. The preparation typically requires the use of solvents and catalysts to facilitate the reaction .
Industrial Production Methods
Industrial production of para-fluorohexahydrosiladifenidol follows similar synthetic routes but on a larger scale. The process involves optimizing reaction conditions to ensure high yield and purity. This may include the use of advanced techniques such as continuous flow reactors and automated systems to maintain consistency and efficiency .
Chemical Reactions Analysis
Types of Reactions
Para-fluorohexahydrosiladifenidol undergoes various chemical reactions, including:
Oxidation: The compound can be oxidized under specific conditions to form corresponding oxides.
Reduction: Reduction reactions can convert the compound into different reduced forms.
Substitution: Substitution reactions, particularly involving the fluorine atom, can lead to the formation of various derivatives.
Common Reagents and Conditions
Oxidation: Common oxidizing agents include potassium permanganate and hydrogen peroxide.
Reduction: Reducing agents such as lithium aluminum hydride and sodium borohydride are often used.
Substitution: Halogenating agents and nucleophiles are commonly employed in substitution reactions.
Major Products Formed
The major products formed from these reactions depend on the specific reagents and conditions used. For example, oxidation may yield oxides, while substitution reactions can produce a variety of fluorinated derivatives .
Comparison with Similar Compounds
Similar Compounds
Hexahydrosiladifenidol (HHSiD): The parent compound of para-fluorohexahydrosiladifenidol, known for its muscarinic receptor antagonistic properties.
4-Diphenylacetoxy-N-methyl piperidine methiodide (4-DAMP): Another muscarinic receptor antagonist with similar properties.
Uniqueness
Para-fluorohexahydrosiladifenidol is unique due to its high selectivity for M3 muscarinic receptors, which distinguishes it from other similar compounds. This selectivity makes it particularly useful in research focused on M3 receptor functions and related therapeutic applications .
Properties
IUPAC Name |
cyclohexyl-(4-fluorophenyl)-hydroxy-(3-piperidin-1-ylpropyl)silane | |
---|---|---|
Source | PubChem | |
URL | https://pubchem.ncbi.nlm.nih.gov | |
Description | Data deposited in or computed by PubChem | |
InChI |
InChI=1S/C20H32FNOSi/c21-18-10-12-20(13-11-18)24(23,19-8-3-1-4-9-19)17-7-16-22-14-5-2-6-15-22/h10-13,19,23H,1-9,14-17H2 | |
Source | PubChem | |
URL | https://pubchem.ncbi.nlm.nih.gov | |
Description | Data deposited in or computed by PubChem | |
InChI Key |
ZNSZQJHTFRQUPD-UHFFFAOYSA-N | |
Source | PubChem | |
URL | https://pubchem.ncbi.nlm.nih.gov | |
Description | Data deposited in or computed by PubChem | |
Canonical SMILES |
C1CCC(CC1)[Si](CCCN2CCCCC2)(C3=CC=C(C=C3)F)O | |
Source | PubChem | |
URL | https://pubchem.ncbi.nlm.nih.gov | |
Description | Data deposited in or computed by PubChem | |
Molecular Formula |
C20H32FNOSi | |
Source | PubChem | |
URL | https://pubchem.ncbi.nlm.nih.gov | |
Description | Data deposited in or computed by PubChem | |
DSSTOX Substance ID |
DTXSID60922113 | |
Record name | Cyclohexyl(4-fluorophenyl)[3-(piperidin-1-yl)propyl]silanol | |
Source | EPA DSSTox | |
URL | https://comptox.epa.gov/dashboard/DTXSID60922113 | |
Description | DSSTox provides a high quality public chemistry resource for supporting improved predictive toxicology. | |
Molecular Weight |
349.6 g/mol | |
Source | PubChem | |
URL | https://pubchem.ncbi.nlm.nih.gov | |
Description | Data deposited in or computed by PubChem | |
CAS No. |
116679-83-5 | |
Record name | 4-Fluorohexahydrosiladifenidol | |
Source | ChemIDplus | |
URL | https://pubchem.ncbi.nlm.nih.gov/substance/?source=chemidplus&sourceid=0116679835 | |
Description | ChemIDplus is a free, web search system that provides access to the structure and nomenclature authority files used for the identification of chemical substances cited in National Library of Medicine (NLM) databases, including the TOXNET system. | |
Record name | Cyclohexyl(4-fluorophenyl)[3-(piperidin-1-yl)propyl]silanol | |
Source | EPA DSSTox | |
URL | https://comptox.epa.gov/dashboard/DTXSID60922113 | |
Description | DSSTox provides a high quality public chemistry resource for supporting improved predictive toxicology. | |
Record name | 4-FLUOROHEXAHYDROSILADIFENIDOL | |
Source | FDA Global Substance Registration System (GSRS) | |
URL | https://gsrs.ncats.nih.gov/ginas/app/beta/substances/XEO1GM7K2V | |
Description | The FDA Global Substance Registration System (GSRS) enables the efficient and accurate exchange of information on what substances are in regulated products. Instead of relying on names, which vary across regulatory domains, countries, and regions, the GSRS knowledge base makes it possible for substances to be defined by standardized, scientific descriptions. | |
Explanation | Unless otherwise noted, the contents of the FDA website (www.fda.gov), both text and graphics, are not copyrighted. They are in the public domain and may be republished, reprinted and otherwise used freely by anyone without the need to obtain permission from FDA. Credit to the U.S. Food and Drug Administration as the source is appreciated but not required. | |
Q1: What is the primary mechanism of action of p-F-HHSiD?
A1: p-F-HHSiD acts as a competitive antagonist at muscarinic receptors. [1, 2, 4, 5, 7-10, 13, 16, 18-21, 23] This means it binds to the receptor, preventing the binding of endogenous agonists like acetylcholine, and thus, inhibiting downstream signaling events.
Q2: Which muscarinic receptor subtypes does p-F-HHSiD primarily target?
A2: While initially characterized as an M1-selective antagonist, [] subsequent studies revealed that p-F-HHSiD demonstrates varying affinities for muscarinic receptor subtypes depending on the tissue or species studied. It consistently shows higher affinity for M3 receptors compared to M2 receptors, but its selectivity for M1 versus M3 can be inconsistent. [1, 2, 4-6, 8-10, 13, 16, 18-21, 23]
Q3: What are the downstream consequences of p-F-HHSiD binding to muscarinic receptors in different tissues?
A3: The effects of p-F-HHSiD vary depending on the muscarinic receptor subtype and the tissue involved:
- Smooth muscle: p-F-HHSiD inhibits acetylcholine-induced contractions in various smooth muscle preparations, including the ileum, [, , , , ] trachea, [, ] oesophagus, [] bladder, [, , ] and blood vessels. [, , , , , ]
- Glands: p-F-HHSiD inhibits acetylcholine-induced secretion in glands, such as salivary glands [] and gastric glands. []
- Central Nervous System: p-F-HHSiD has been implicated in altering sleep architecture, particularly affecting slow-wave sleep. [, ]
Q4: What is the molecular formula and weight of p-F-HHSiD?
A4: Unfortunately, this specific information is not provided in the set of scientific papers provided.
Q5: Is there any spectroscopic data available for p-F-HHSiD?
A5: The provided research papers do not delve into detailed spectroscopic characterization of p-F-HHSiD.
Q6: How does the sila-substitution in p-F-HHSiD (compared to its carbon analog) affect its muscarinic receptor affinity?
A6: Studies comparing p-F-HHSiD to its carbon analog, p-F-HHD, demonstrate that sila-substitution generally enhances affinity for all muscarinic receptor subtypes, particularly M3 and M5. []
Q7: What is the impact of modifying the cyclic amino group in p-F-HHSiD on its antimuscarinic properties?
A7: Replacing the piperidine ring of p-F-HHSiD with pyrrolidine or hexamethylenimine moieties leads to compounds with higher affinity for M1 and M3 receptors but lower affinity for M2 receptors. This modification shifts the selectivity profile to M1/M3 > M2, similar to p-F-HHD. []
Q8: How does quaternization of the piperidine nitrogen in p-F-HHSiD influence its receptor subtype selectivity?
A8: Quaternization of p-F-HHSiD, yielding its methiodide derivative, significantly increases affinity for M1 receptors while reducing affinity for M2 and M3 receptors. [] This modification enhances M1 selectivity.
Disclaimer and Information on In-Vitro Research Products
Please be aware that all articles and product information presented on BenchChem are intended solely for informational purposes. The products available for purchase on BenchChem are specifically designed for in-vitro studies, which are conducted outside of living organisms. In-vitro studies, derived from the Latin term "in glass," involve experiments performed in controlled laboratory settings using cells or tissues. It is important to note that these products are not categorized as medicines or drugs, and they have not received approval from the FDA for the prevention, treatment, or cure of any medical condition, ailment, or disease. We must emphasize that any form of bodily introduction of these products into humans or animals is strictly prohibited by law. It is essential to adhere to these guidelines to ensure compliance with legal and ethical standards in research and experimentation.