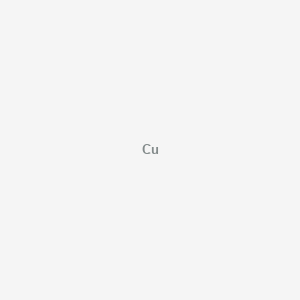
Copper
Overview
Description
Copper(2+) is an ion of this compound carrying a double positive charge. It has a role as a cofactor. It is a divalent metal cation, a this compound cation and a monoatomic dication.
This compound(2+) is a metabolite found in or produced by Saccharomyces cerevisiae.
See also: Cupric Sulfate (active moiety of); Cupric Chloride (active moiety of); this compound Gluconate (is active moiety of) ... View More ...
Mechanism of Action
Target of Action
Copper (Cu) is an essential trace element that plays a crucial role in various physiological processes within cells . It acts as a co-factor for many enzymes, including cytochrome c oxidase, monoamine oxidase, and superoxide dismutase . The primary intracellular targets of this compound toxicity are the iron-sulfur clusters of dehydratases .
Mode of Action
This compound is absorbed from the gut via high-affinity this compound uptake protein and likely through low-affinity this compound uptake protein and natural resistance-associated macrophage protein-2 . It is believed that this compound is reduced to the Cu1+ form prior to transport . Once inside the cell, it is bound to this compound transport protein ATOX1, which shuttles the ion to this compound transporting ATPase-1 on the golgi membrane . This compound ions are known to reduce fertility when released from this compound-containing intrauterine devices .
Biochemical Pathways
This compound participates in many biochemical pathways. It is an essential cofactor for oxidase enzymes that catalyze oxidation-reduction reactions in various metabolic pathways . These this compound-dependent enzymes, or cuproenzymes, participate in energy (ATP) production, iron metabolism, connective tissue formation, and neurotransmission . This compound is also involved in a wide spectrum of proteins, such as this compound/zinc superoxide dismutase (Cu/Zn SOD or SOD1), cytochrome c oxidase (C c O), mitogen-activated protein kinase MEK1 .
Pharmacokinetics (ADME Properties)
The pharmacokinetics of this compound involve its absorption, distribution, metabolism, and excretion (ADME). This compound is absorbed from the gut and transported into the blood. It arrives at the liver through the portal vein and is incorporated into hepatocytes . This compound can then be secreted into the bile or the blood via the Atox1/ATP7B/ceruloplasmin route . In the bloodstream, this micronutrient can reach peripheral tissues and is again incorporated by Ctr1 .
Result of Action
The molecular and cellular effects of this compound’s action are significant. This compound can trigger a specific form of cell death, namely, cuproptosis, which is triggered by excessive levels of intracellular this compound . Cuproptosis induces the aggregation of mitochondrial lipoylated proteins, and the loss of iron-sulfur cluster proteins . An excess of this compound ions in cells can generate free radicals and increase oxidative stress .
Action Environment
The action, efficacy, and stability of this compound are influenced by various environmental factors. This compound’s effect in water, sediment, and soil depends largely on the chemical and physical characteristics of the local environment, as well as the bioavailability of different chemical forms of this compound to organisms in the environment .
Biochemical Analysis
Biochemical Properties
Copper serves as a constituent element or enzyme cofactor that participates in many biochemical pathways . It plays a key role in photosynthesis, respiration, ethylene sensing, and antioxidant systems . This compound ions readily form complexes with biomolecules containing amino acid residues .
Cellular Effects
This compound can trigger a specific form of cell death, namely, cuproptosis, which is triggered by excessive levels of intracellular this compound . Cuproptosis induces the aggregation of mitochondrial lipoylated proteins, and the loss of iron-sulfur cluster proteins . In neurodegenerative diseases, the pathogenesis and progression of neurological disorders are linked to this compound homeostasis .
Molecular Mechanism
This compound metabolism involves uptake, distribution, sequestration, and excretion, at both the cellular and systemic levels . Mammalian enterocytes take in bioavailable this compound ions from the diet in a Ctr1-dependent manner . After incorporation, cuprous ions are delivered to ATP7A, which pumps Cu+ from enterocytes into the blood .
Temporal Effects in Laboratory Settings
This compound plays vital roles in numerous cellular processes and its imbalance can lead to oxidative stress and dysfunction . Recent research has unveiled a unique form of this compound-induced cell death, termed cuproptosis, which differs from known cell death mechanisms .
Dosage Effects in Animal Models
Chronic this compound toxicity has been shown to cause hepatotoxicity and liver cirrhosis as observed in Wilson’s disease . Substantial evidence accrued over time have shown considerable increase in animal studies demonstrating Alzheimer’s disease like pathology due to chronic this compound-intoxication under certain conditions .
Metabolic Pathways
This compound is involved in important biochemical processes, such as homeostasis, iron transport, respiration, and metabolism, as a result of its redox abilities in the biological environment . It is involved in the formation of hemoglobin, melanin, myelin, and thyroxine and are constituents of enzymes such as lysyl oxidaze, ceruloplasmin, superoxide dismutase, and cytochrome c-oxidase .
Subcellular Localization
This compound ions have been found in cell walls, plasmodesmata, and within the nuclei and chloroplasts . In peripheral tissue cells, cuprous ions are either sequestrated by molecules such as metallothioneins or targeted to utilization pathways by chaperons such as Atox1, Cox17, and CCS .
Properties
CAS No. |
7440-50-8 |
---|---|
Molecular Formula |
Cu+2 |
Molecular Weight |
63.55 g/mol |
IUPAC Name |
copper(2+) |
InChI |
InChI=1S/Cu/q+2 |
InChI Key |
JPVYNHNXODAKFH-UHFFFAOYSA-N |
impurities |
/In electrolytic copper/ the highest level of impurities other than oxygen are found only to the extent of 15-30 ppm. Up to 0.05% oxygen is present in the form of copper(I) oxide. Average impurity levels: Antimony, 3.41 ppm; arsenic, 1.39 ppm; bismuth, 0.36 ppm; iron, 6.07 ppm; lead, 4.08 ppm; oxygen 327.25 ppm; nickel, 3.41 ppm; selenium, 1.10 ppm; silver, 11.19 ppm; sulfur, 10.00 ppm; tellurium, 1.16 ppm; tin, 1.63 ppm. |
SMILES |
[Cu] |
Canonical SMILES |
[Cu+2] |
boiling_point |
4703 °F at 760 mmHg (NIOSH, 2024) 2595 °C 4703 °F |
Color/Form |
Reddish, lustrous, ductile, malleable metal Red metal; cubic |
density |
8.94 (NIOSH, 2024) - Denser than water; will sink 8.94 Relative density (water = 1): 8.9 |
melting_point |
1083 °C |
7440-50-8 7440-50-8 (copper); 1317-38-0 (copper oxide) |
|
physical_description |
Solid |
Pictograms |
Acute Toxic; Irritant; Environmental Hazard |
shelf_life |
BECOMES DULL WHEN EXPOSED TO AIR. IN MOIST AIR GRADUALLY BECOMES COATED WITH GREEN BASIC CARBONATE. |
solubility |
Insoluble (NIOSH, 2024) 8.96g/mL Slightly sol in dilute acid Slowly soluble in ammonia water Solubility in water: none Insoluble |
Synonyms |
GRAPHIMET CU-5; C.I. 77400; COPPER IN GRAPHITE; COPPER, NATURAL; COPPER, OIL BASED STANDARD SOLUTION; COPPER METALLO-ORGANIC STANDARD; COPPER LOW RANGE; COPPER METAL |
vapor_pressure |
0 mmHg (approx) (NIOSH, 2024) 1 mm Hg @ 1628 °C 0 mmHg (approx) |
Origin of Product |
United States |
Synthesis routes and methods I
Procedure details
Synthesis routes and methods II
Procedure details
Synthesis routes and methods III
Procedure details
Synthesis routes and methods IV
Procedure details
Q1: How does copper affect algae, specifically Chlorella pyrenoidosa?
A: this compound exposure, especially in combination with other metals like cadmium, inhibits the growth of Chlorella pyrenoidosa []. This inhibition is linked to the accumulation of this compound in the algae's metal-rich granules and heat-stable proteins, affecting their subcellular distribution and overall growth [].
Q2: Can this compound supplementation impact this compound levels in animals?
A: Yes, administering this compound nanoparticles or this compound sulfate to broiler chickens in ovo (into the egg) leads to increased this compound accumulation in organs like the liver and spleen []. This suggests that direct this compound supplementation can influence this compound levels in animal tissues [].
Q3: What is the role of the CCS1 protein in this compound homeostasis in Arabidopsis?
A: The CCS1 protein acts as a this compound chaperone, delivering this compound to CSD1 and CSD2, two crucial enzymes for mitigating oxidative stress in Arabidopsis []. The expression of CCS1, and consequently the activity of CSDs, is regulated by microRNA398 (miR398), highlighting a complex interplay between this compound availability and gene expression [].
Q4: What role does the prion protein (PrPc) play in this compound uptake and release in cerebellar cells?
A: Research suggests that PrPc facilitates this compound uptake by cerebellar cells, and this uptake depends on the presence of histidine residues []. Moreover, PrPc seems to be involved in the veratridine-induced release of this compound at synapses, possibly indicating a role in neurotransmission [].
Q5: What is the molecular formula, weight, and spectroscopic data for this compound (II) oxide (CuO)?
A: While not explicitly stated in the provided research, CuO has a molecular weight of 79.545 g/mol. Spectroscopically, CuO exhibits characteristic peaks in techniques like X-ray diffraction (XRD) used to identify its presence in materials [, ].
Q6: How does this compound interact with silicon carbide (SiC) during the formation of this compound-SiC composites?
A: During mechanical alloying in a planetary activator, this compound and SiC particles interact to form a "puff-pastry" structure []. This unique structure arises from the repeated fracturing and welding of this compound particles, eventually leading to a homogenous distribution of SiC within the this compound matrix [].
Q7: How does this compound slag affect the properties of concrete when used as a partial replacement for fine aggregate?
A: Replacing a portion of fine aggregate with this compound slag in concrete mixes, particularly at 20% by weight, can impact its mechanical characteristics. Studies are exploring its effect on compressive strength, split tensile strength, and overall workability in concrete applications [, ].
Q8: Can this compound slag be combined with other materials to enhance concrete properties?
A: Yes, research indicates that using this compound slag with dolomite powder, a magnesium-rich material, can influence concrete properties. Studies are investigating the combined effect of partially replacing cement with dolomite and fine aggregate with this compound slag on the strength and workability of concrete [].
Q9: How does the surface composition of this compound affect the performance of no-flow underfill materials in electronics?
A: The presence of this compound oxide (CuO) on this compound pads can hinder the wetting of solder bumps during the reflow process with no-flow underfill materials []. This highlights the importance of surface cleanliness and composition for optimal adhesion and performance in electronic packaging applications [].
Q10: How does the addition of this compound affect the electrical conductivity of Pb-Sb alloys?
A: Increasing the this compound content in Pb-Sb alloys generally enhances their electrical conductivity []. This improvement is attributed to the influence of this compound on the alloy's melting temperature and the resulting changes in electron mobility within the material [].
Q11: How do silver and this compound coatings affect the properties of diamond and graphite reinforced this compound composites?
A: Applying silver or this compound coatings to diamond and graphite reinforcements before incorporating them into this compound matrices can influence the final composite's microstructure and properties []. This coating technique can improve particle distribution and enhance thermal conductivity, making these composites potentially suitable for heat sink applications [].
Q12: How is this compound extracted from mining industry recirculation waters?
A: Selective extraction of this compound from wastewater, particularly in the presence of other metals like zinc, can be achieved using reagent-based techniques. One method involves sulphidation in an acidic pH range, employing a sulphur solution in sodium hydroxide to precipitate this compound as sulphides []. This process yields a high-concentration this compound concentrate, allowing for efficient recovery of this compound from complex wastewater streams [].
Q13: What is reduction-roast leaching, and how is it used to extract this compound from converter slag?
A: Reduction-roasting is a pretreatment step employed to enhance this compound recovery from converter slag, which often contains this compound in forms that are difficult to leach directly. Roasting with a reducing agent like non-coking coal converts this compound compounds into more leachable forms, facilitating subsequent leaching with lixiviants like acetic acid to extract this compound more efficiently [].
Q14: Can organic acids be used to leach this compound and cobalt from solutions, and how are these metals separated?
A: Yes, organic acids can effectively leach this compound and cobalt from solutions. Solvent extraction offers a method for separating these metals. By using specific extractants like LIX 84 for this compound and Cyanex 272 or Versatic acid 10 for cobalt, these metals can be selectively extracted and separated based on differences in their extraction pH and affinity for the extractants [].
Q15: How are this compound nanoparticles used in modifying phenolic resins?
A: Incorporating this compound nanoparticles into phenolic resins during the synthesis process can significantly enhance the resin's properties []. The presence of this compound nanoparticles improves the resin's thermal stability at lower temperatures, increases its impact strength, and reduces its brittleness, making it more suitable for applications requiring enhanced mechanical properties [].
Q16: What is the process of fabricating nanotwinned this compound redistribution wires, and what are their advantages?
A: Nanotwinned this compound redistribution wires are fabricated using a process that involves seed layer formation, photoresist patterning, and pulse electroplating []. The pulse electroplating, combined with rapid annealing, promotes the formation of high-density nanotwins within the this compound structure []. These nanotwinned wires exhibit superior mechanical properties, allowing for reduced wire size and improved thermal-mechanical reliability in microelectronic applications [].
Q17: What are this compound nitrilotriacetate (this compound-NTA)'s effects on genetic this compound metabolism disorders?
A: Studies in mice with the crinkled (cr) gene mutation, a model for Menkes' syndrome (a human genetic this compound disorder), show that this compound-NTA supplementation is more effective than this compound sulfate in increasing survival rates and improving this compound levels []. This suggests that this compound-NTA could be a potential therapeutic agent for treating genetic this compound deficiency disorders [].
Q18: Can this compound drum culture be integrated into primary school art education, and what are the benefits?
A: Yes, integrating this compound drum culture into primary school art classrooms, particularly in regions where this cultural heritage is significant, can provide numerous benefits. It offers opportunities for students to learn about local traditions, explore the artistic elements of this compound drums, and develop a sense of cultural identity and appreciation [].
Q19: What is the historical significance of this compound in the context of bronze vessels from the Shang and Zhou Dynasties in China?
A: Analysis of trace elements in ancient bronze vessels provides insights into the provenance of this compound used in their production. By comparing these trace element profiles with those of this compound ores from known mining sites of that era, researchers can trace the origin of the this compound and understand ancient trade routes and material exchange networks [].
Q20: What is the structure of chloro(N,N-diethylglycinato)this compound(II)?
A: Chloro(N,N-diethylglycinato)this compound(II) forms a one-dimensional polymer chain where this compound atoms are bridged by carboxylate groups of the N,N-diethylglycinato ligand []. The this compound atom exhibits a distorted five-coordination geometry, surrounded by three carboxylato oxygen atoms, one nitrogen atom, and one chlorine atom [].
Q21: How are this compound oxycarbonates relevant in high-Tc superconductor research?
A: this compound oxycarbonates with layered structures have emerged as a new class of high-Tc superconductors. The partial replacement of CuO polyhedra with carbonate groups in these materials enhances their two-dimensional structural character, a key factor contributing to their superconducting properties [].
Q22: How is polyurea resin used to remove this compound (II) ions from aqueous solutions?
A: Polyurea resin, specifically polyamine-polyurea chelating resin, exhibits a high adsorption capacity for this compound (II) ions in aqueous solutions []. This adsorption process is influenced by factors like pH, adsorbent dosage, contact time, and initial metal concentration. The experimental data often fit well with the Langmuir and Freundlich isotherm models, providing insights into the adsorption mechanism [].
Q23: How does the wood preservative chromated-copper-arsenate (CCA) affect the biodegradation of pentachlorophenol (PCP)?
A: CCA inhibits the bacterial degradation of PCP, as demonstrated by its effect on Flavobacterium sp. strain ATCC 53874 []. The presence of CCA increases the lag time for PCP degradation and extends the time for complete degradation. This inhibitory effect is observed even at very low concentrations of CCA components, highlighting the potential environmental impact of CCA on bioremediation processes [].
Q24: What are the environmental concerns related to this compound, and how can its impact be mitigated?
A: While this compound is an essential micronutrient, excessive this compound levels can be detrimental to ecosystems. this compound released from industrial activities can contaminate soil and water resources, adversely affecting aquatic life and potentially accumulating in the food chain [, ]. Mitigation strategies include implementing stricter regulations on industrial discharge, developing efficient wastewater treatment technologies to remove this compound, and exploring alternative materials with lower environmental impact [].
Q25: What are the safety regulations surrounding the handling and disposal of this compound-containing materials?
A: Safe handling and disposal of this compound-containing materials are crucial to minimize risks to human health and the environment. Regulations and guidelines vary depending on the specific compound and its intended use. General safety measures include wearing appropriate personal protective equipment, ensuring proper ventilation in work areas, and adhering to established protocols for waste disposal [].
Q26: How can this compound resources be managed more sustainably?
A: Sustainable this compound resource management requires a multi-faceted approach encompassing efficient extraction and processing methods, promoting recycling and reuse, and developing substitutes when feasible []. Investing in research to improve the efficiency of this compound recycling processes and exploring the use of alternative materials in applications where this compound is currently dominant are essential for ensuring long-term sustainability [].
Q27: What are the promising areas for future research related to this compound?
A: Future research avenues include: * Developing new this compound-based materials with enhanced properties for applications in electronics, energy, and catalysis [, ].* Understanding the intricate mechanisms of this compound homeostasis in biological systems and its implications for human health and disease [, , ]. * Designing innovative and environmentally friendly approaches for this compound extraction, recycling, and waste management to minimize its ecological footprint [].
Disclaimer and Information on In-Vitro Research Products
Please be aware that all articles and product information presented on BenchChem are intended solely for informational purposes. The products available for purchase on BenchChem are specifically designed for in-vitro studies, which are conducted outside of living organisms. In-vitro studies, derived from the Latin term "in glass," involve experiments performed in controlled laboratory settings using cells or tissues. It is important to note that these products are not categorized as medicines or drugs, and they have not received approval from the FDA for the prevention, treatment, or cure of any medical condition, ailment, or disease. We must emphasize that any form of bodily introduction of these products into humans or animals is strictly prohibited by law. It is essential to adhere to these guidelines to ensure compliance with legal and ethical standards in research and experimentation.