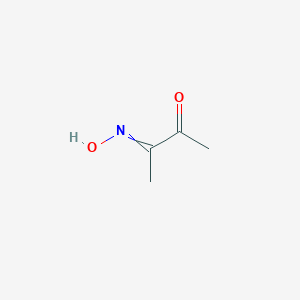
2,3-Butanedione monoxime
Overview
Description
2,3-Butanedione monoxime, also known as diacetyl monoxime, is a chemical compound with the molecular formula C₄H₇NO₂. It is a colorless solid that is primarily known for its role as a non-competitive inhibitor of myosin ATPase activity. This compound has significant applications in various fields, including chemistry, biology, and medicine .
Mechanism of Action
Target of Action
The primary target of 2,3-Butanedione Monoxime (BDM) is skeletal muscle myosin-II . Myosin-II plays a crucial role in muscle contraction, and its interaction with actin is fundamental to this process .
Mode of Action
BDM is a well-characterized, low-affinity, non-competitive inhibitor of skeletal muscle myosin-II . BDM enhances the rigidity of myosin binding to actin, which can slow down the transition of actomyosin from the A∙M∙ADP∙Pi state to the A∙M∙ADP state . This is one of the reasons for the decrease in the ATPase activity of myosin .
Biochemical Pathways
BDM affects the actin-myosin interaction, a key component of the muscle contraction pathway .
Pharmacokinetics
It’s known that bdm is used at millimolar concentrations in cell biological experiments .
Result of Action
BDM’s inhibition of myosin-II ATPase activity results in a decrease in muscle contraction . It also induces ion and fluid transport in MDCK monolayers . BDM can be used to increase the efficiency of myosin conformational rearrangements in the presence of tropomyosin with the R90P mutation associated with congenital myopathy .
Action Environment
The action of BDM can be influenced by environmental factors such as the presence of other proteins and ions. For example, in the absence of the regulatory proteins tropomyosin and troponin, BDM enhances the rigidity of myosin binding to actin . Also, replacement of extracellular Na+ and Cl- and inhibition of Na±K±ATPase can block the induction of domes, a result of BDM’s action .
Biochemical Analysis
Biochemical Properties
2,3-Butanedione monoxime plays a significant role in biochemical reactions. It interacts with myosin-II, a protein involved in muscle contraction . BDM acts as a non-competitive inhibitor of myosin ATPase activity . It does not inhibit the ATPase activity of other myosins .
Cellular Effects
This compound has notable effects on various types of cells and cellular processes. For instance, in epithelial MDCK cell monolayers, cells exposed to BDM showed a remarkable increase in the number of domes . This suggests that BDM influences cell function by modulating cell shape and polarity .
Molecular Mechanism
The molecular mechanism of this compound involves its interaction with myosin-II. BDM inhibits the ATPase activity of myosin-II, thereby affecting the actin-myosin interaction . This interaction is crucial for muscle contraction, suggesting that BDM exerts its effects at the molecular level by modulating this process .
Temporal Effects in Laboratory Settings
In laboratory settings, the effects of this compound change over time. For instance, when myotubes cultured in BDM-containing media were transferred to control media, sarcomeric structures were formed in 2–3 days . This suggests that the inhibitory effect of BDM on myotubes is reversible .
Dosage Effects in Animal Models
In animal models, the effects of this compound vary with different dosages. For example, in a pig model of cardiac arrest, increasing the dose of BDM improved the hemodynamic effectiveness of cardiopulmonary resuscitation (CPR), thus ultimately improving resuscitability .
Metabolic Pathways
This compound is involved in metabolic pathways related to muscle contraction. It interacts with myosin-II, an enzyme crucial for muscle contraction
Transport and Distribution
Given its role as a myosin-II inhibitor, it is likely that it interacts with proteins involved in muscle contraction .
Subcellular Localization
Given its role in inhibiting myosin-II, it is likely localized to areas where myosin-II is present, such as muscle fibers
Preparation Methods
Synthetic Routes and Reaction Conditions: 2,3-Butanedione monoxime can be synthesized through the reaction of diacetyl (2,3-butanedione) with hydroxylamine. The reaction typically involves the following steps:
Reactants: Diacetyl and hydroxylamine.
Conditions: The reaction is carried out in an aqueous medium, often with the addition of a base such as sodium hydroxide to facilitate the reaction.
Procedure: Diacetyl is mixed with hydroxylamine in water, and the mixture is stirred at room temperature.
Industrial Production Methods: In industrial settings, the production of this compound follows a similar synthetic route but on a larger scale. The reaction conditions are optimized to ensure high yield and purity. The compound is typically produced in batch reactors, and the product is purified through recrystallization or distillation .
Chemical Reactions Analysis
Types of Reactions: 2,3-Butanedione monoxime undergoes various chemical reactions, including:
Oxidation: It can be oxidized to form corresponding oximes and other derivatives.
Reduction: The compound can be reduced to form amines and other reduced products.
Substitution: It can participate in nucleophilic substitution reactions, where the oxime group is replaced by other nucleophiles.
Common Reagents and Conditions:
Oxidation: Common oxidizing agents include potassium permanganate and hydrogen peroxide.
Reduction: Reducing agents such as sodium borohydride and lithium aluminum hydride are used.
Substitution: Nucleophiles like amines and thiols are commonly used in substitution reactions.
Major Products:
Oxidation: Oxidized derivatives of this compound.
Reduction: Amines and other reduced compounds.
Substitution: Various substituted oxime derivatives.
Scientific Research Applications
2,3-Butanedione monoxime has a wide range of applications in scientific research:
Chemistry: It is used as a reagent for the colorimetric determination of urea and other compounds.
Biology: The compound is used to study the actin-myosin interaction in muscle fibers.
Medicine: this compound is investigated for its potential therapeutic applications in treating muscle disorders and cardiomyopathies. .
Comparison with Similar Compounds
Diacetyl monoxime: A closely related compound with similar chemical properties.
Biacetyl monoxime: Another similar compound with comparable applications and mechanisms of action.
Uniqueness: 2,3-Butanedione monoxime is unique in its ability to inhibit myosin ATPase activity non-competitively. This property makes it particularly useful in studying muscle physiology and developing therapeutic agents for muscle-related disorders .
Properties
CAS No. |
57-71-6 |
---|---|
Molecular Formula |
C4H7NO2 |
Molecular Weight |
101.10 g/mol |
IUPAC Name |
(3Z)-3-hydroxyiminobutan-2-one |
InChI |
InChI=1S/C4H7NO2/c1-3(5-7)4(2)6/h7H,1-2H3/b5-3- |
InChI Key |
FSEUPUDHEBLWJY-HYXAFXHYSA-N |
SMILES |
CC(=NO)C(=O)C |
Isomeric SMILES |
C/C(=N/O)/C(=O)C |
Canonical SMILES |
CC(=NO)C(=O)C |
Appearance |
A crystalline solid |
boiling_point |
365 to 367 °F at 760 mmHg (NTP, 1992) |
melting_point |
171 to 172 °F (NTP, 1992) |
Key on ui other cas no. |
57-71-6 17019-25-9 |
physical_description |
2-oxime 2,3-butanedione is a cream-colored powder. (NTP, 1992) Cream-colored solid; [CAMEO] White crystalline powder; [Alfa Aesar MSDS] |
Pictograms |
Irritant |
solubility |
10 to 50 mg/mL at 64 °F (NTP, 1992) |
Synonyms |
2,3-BDMO 2,3-butanedione monooxime 2,3-butanedione monoxime butanedione monoxime diacetyl monooxime diacetyl monoxime diacetylmonoxime KBDO potassium 2,3-butanedione monoximate |
Origin of Product |
United States |
Retrosynthesis Analysis
AI-Powered Synthesis Planning: Our tool employs the Template_relevance Pistachio, Template_relevance Bkms_metabolic, Template_relevance Pistachio_ringbreaker, Template_relevance Reaxys, Template_relevance Reaxys_biocatalysis model, leveraging a vast database of chemical reactions to predict feasible synthetic routes.
One-Step Synthesis Focus: Specifically designed for one-step synthesis, it provides concise and direct routes for your target compounds, streamlining the synthesis process.
Accurate Predictions: Utilizing the extensive PISTACHIO, BKMS_METABOLIC, PISTACHIO_RINGBREAKER, REAXYS, REAXYS_BIOCATALYSIS database, our tool offers high-accuracy predictions, reflecting the latest in chemical research and data.
Strategy Settings
Precursor scoring | Relevance Heuristic |
---|---|
Min. plausibility | 0.01 |
Model | Template_relevance |
Template Set | Pistachio/Bkms_metabolic/Pistachio_ringbreaker/Reaxys/Reaxys_biocatalysis |
Top-N result to add to graph | 6 |
Feasible Synthetic Routes
A: BDM primarily interacts with the myosin ATPase, a key enzyme in muscle contraction. [] It acts as an uncompetitive inhibitor, specifically targeting the M.ADP.Pi complex. [] This interaction reduces the concentration of the "strong" actin-binding state (M*.ADP) and increases the concentration of the "weak" binding state (M.ADP.Pi). [] This ultimately leads to inhibition of force production in muscle. [, ] Additionally, BDM can affect intracellular Ca2+ handling, further contributing to its negative inotropic effects. [, ]
ANone:
A: BDM possesses "chemical phosphatase" activity. [, ] This property allows it to dephosphorylate acetylcholinesterase inhibited by organophosphates. [, ] This reactivation ability makes BDM a potential therapeutic agent for organophosphate poisoning. []
A: While the provided abstracts don't delve into detailed SAR studies for BDM, research on related oximes, particularly in the context of organophosphate poisoning, suggests that structural modifications can significantly impact their reactivation potency and selectivity towards different enzymes. [] For example, uncharged oximes, like BDM, tend to be better reactivators of carboxylesterase compared to cationic oximes, highlighting the importance of charge in target selectivity. []
A: Numerous studies demonstrate BDM's efficacy in various in vitro and ex vivo settings. [, , , , , , , , , , , , , , , , , , , , , , , , , , , , , ] For instance, BDM improves the preservation of isolated rat hearts during hypothermic storage. [, ] It also protects against reperfusion injury in isolated heart models. [, ] In vivo studies are limited, but one study suggests BDM's potential to protect against oxidative stress in a rat model. []
ANone: The provided abstracts don't discuss resistance mechanisms or cross-resistance related to BDM. This aspect would require further investigation.
A: While the provided abstracts primarily focus on BDM's effects on muscle contraction and its potential therapeutic benefits, some studies indicate potential toxicity. One abstract specifically mentions "2,3-butanedione monoxime poisoning." [] Additionally, research on its effects on various ion channels and cellular processes suggests the possibility of off-target effects. [, , ] Therefore, careful consideration of potential toxicity and long-term effects is crucial when using or investigating BDM.
Disclaimer and Information on In-Vitro Research Products
Please be aware that all articles and product information presented on BenchChem are intended solely for informational purposes. The products available for purchase on BenchChem are specifically designed for in-vitro studies, which are conducted outside of living organisms. In-vitro studies, derived from the Latin term "in glass," involve experiments performed in controlled laboratory settings using cells or tissues. It is important to note that these products are not categorized as medicines or drugs, and they have not received approval from the FDA for the prevention, treatment, or cure of any medical condition, ailment, or disease. We must emphasize that any form of bodily introduction of these products into humans or animals is strictly prohibited by law. It is essential to adhere to these guidelines to ensure compliance with legal and ethical standards in research and experimentation.