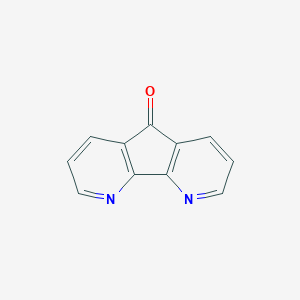
4,5-Diazafluoren-9-one
Overview
Description
4,5-Diazafluoren-9-one is a heterocyclic compound with the molecular formula C11H6N2O. It is a derivative of fluorenone and contains two nitrogen atoms in the five-membered ring. This compound is known for its use as a building block in the synthesis of various heterocyclic compounds and as a fluorescent probe .
Mechanism of Action
Target of Action
4,5-Diazafluoren-9-one is a heterocyclic compound that behaves like cyclopentadienones . It reacts with [CpCo (C2H4)2] to form air-stable sandwich-type complexes [CpCo (η4-2)] . This suggests that the primary targets of this compound are metal ions, particularly cobalt ions.
Mode of Action
The compound interacts with its targets by forming stable complexes. The unshared electron pairs located within the molecular backbone of this compound can act cooperatively to bind transition metal cations . This interaction results in the formation of stable complexes, which could potentially influence the biochemical pathways in which these metal ions are involved.
Pharmacokinetics
It is known to be soluble in tetrahydrofuran (thf), dichloromethane, benzene, and toluene This suggests that the compound may have good bioavailability in systems where these solvents are present
Action Environment
Environmental factors can influence the action, efficacy, and stability of this compound. For instance, the solubility of the compound in various solvents suggests that the presence of these solvents in the environment could influence its action and efficacy . Additionally, the compound’s ability to form air-stable complexes suggests that it may have good stability in various environmental conditions .
Biochemical Analysis
Biochemical Properties
4,5-Diazafluoren-9-one has been used in the preparation of heterocyclic ligands by condensation with various diamines, hydrazine, 1,4-phenylenediamine, benzidine, and 4,4′-methylenedianiline . The nature of these interactions involves the formation of new bonds between the nitrogen atoms in this compound and the other molecules .
Cellular Effects
Given its role in the synthesis of various heterocyclic compounds, it can be inferred that it may influence cellular processes indirectly through these compounds .
Molecular Mechanism
It is known to react with [Cp*Co (C2H4)2] to form air-stable sandwich-type complexes . This suggests that it may interact with biomolecules through similar mechanisms.
Temporal Effects in Laboratory Settings
It is known to be soluble in various solvents such as THF, benzene, dichloromethane, and toluene , which suggests that it may be stable in these solvents over time.
Metabolic Pathways
Given its role in the synthesis of various heterocyclic compounds, it may be involved in the metabolic pathways of these compounds .
Preparation Methods
Synthetic Routes and Reaction Conditions: 4,5-Diazafluoren-9-one can be synthesized through the condensation of fluorenone with hydrazine or other diamines. The reaction typically involves heating the reactants in the presence of a suitable solvent such as ethanol or acetic acid .
Industrial Production Methods: In industrial settings, the synthesis of this compound may involve more efficient and scalable methods, such as continuous flow synthesis. This approach allows for better control over reaction conditions and yields .
Chemical Reactions Analysis
Types of Reactions: 4,5-Diazafluoren-9-one undergoes various types of chemical reactions, including:
Oxidation: It can be oxidized to form 9-diazo-4,5-diazafluorene.
Reduction: Reduction reactions can convert it into corresponding amines or hydrazines.
Substitution: It can undergo nucleophilic substitution reactions with various nucleophiles.
Common Reagents and Conditions:
Oxidation: Common oxidizing agents include potassium permanganate and hydrogen peroxide.
Reduction: Reducing agents such as sodium borohydride or lithium aluminum hydride are often used.
Substitution: Nucleophiles like amines, hydrazines, and thiols can react with this compound under mild conditions.
Major Products Formed:
Oxidation: 9-diazo-4,5-diazafluorene.
Reduction: Corresponding amines or hydrazines.
Substitution: Various substituted derivatives depending on the nucleophile used.
Scientific Research Applications
4,5-Diazafluoren-9-one has a wide range of applications in scientific research:
Chemistry: It is used as a building block for synthesizing heterocyclic ligands and fluorescent probes.
Biology: Its fluorescent properties make it useful in biological imaging and as a marker in various assays.
Medicine: Research is ongoing into its potential use in drug development and as a diagnostic tool.
Comparison with Similar Compounds
4,5-Diazafluoren-9-one can be compared with other similar compounds such as:
- 1,10-Phenanthroline-5,6-dione
- 1,8-Diazafluoren-9-one
- 9-Fluorenone
- 9,10-Phenanthrenequinone
Uniqueness: this compound is unique due to its dual nitrogen atoms in the five-membered ring, which imparts distinct electronic and fluorescent properties compared to its analogs .
Biological Activity
4,5-Diazafluoren-9-one, commonly referred to as dafone, is a nitrogen-containing heterocyclic compound that has garnered attention in medicinal chemistry due to its diverse biological activities. This article synthesizes findings from various studies focusing on the biological activity of dafone, particularly its antibacterial and anticancer properties, as well as its potential applications in drug delivery systems.
This compound can be synthesized from 1,10-phenanthroline through a series of chemical reactions involving potassium hydroxide (KOH) and other reagents. The compound exhibits significant coordination chemistry, forming complexes with various metal ions such as silver and chromium, which enhance its biological activity.
Biological Activity Overview
Dafone has been studied for multiple biological activities:
-
Antibacterial Activity :
- In a study assessing the antibacterial efficacy of bis-4,5-diazafluoren-9-one silver(I) nitrate against clinical isolates, dafone demonstrated broad-spectrum activity against both Gram-positive and Gram-negative bacteria. Notably, it showed minimal inhibitory concentrations (MIC) of 4 µg/ml against Staphylococcus aureus and Proteus mirabilis, outperforming several conventional antibiotics .
- The compound was also incorporated into hydrogels for potential wound dressing applications. The hydrogels containing dafone exhibited significant zones of inhibition against Pseudomonas aeruginosa, indicating effective antibacterial properties .
-
Anticancer Activity :
- Research has indicated that dafone exhibits cytotoxic effects against various cancer cell lines, including human lung adenocarcinoma (A549) and oral squamous carcinoma (KB). The anti-proliferative effects were evaluated using the MTT assay, revealing that dafone could inhibit cell growth effectively .
- The mechanism of action appears to involve DNA binding, where dafone interacts with DNA through groove binding modes, which may disrupt cellular processes leading to apoptosis in cancer cells .
Antibacterial Efficacy
A detailed study evaluated the antibacterial activity of dafone in comparison with 15 known antibiotics. The results highlighted:
Bacterial Strain | MIC (µg/ml) |
---|---|
Staphylococcus aureus | 4 |
Streptococcus pyogenes | 6 |
Pseudomonas aeruginosa | 8 |
Klebsiella pneumoniae | 6 |
Proteus mirabilis | 4 |
Salmonella sp. | 10 |
This table illustrates the potent antibacterial properties of dafone against clinically relevant pathogens .
Anticancer Studies
In anticancer research involving dafone:
- The cytotoxicity was assessed on A549 and KB cell lines with IC50 values indicating significant potency.
- Flow cytometry analyses revealed that treatment with dafone led to increased apoptosis rates in treated cells compared to controls.
The biological activity of dafone is attributed to its ability to form stable complexes with metal ions and its interaction with cellular macromolecules such as DNA. Studies using circular dichroism (CD) and viscosity measurements suggest that dafone binds to DNA in a groove-binding manner, which could interfere with replication and transcription processes .
Properties
IUPAC Name |
3,13-diazatricyclo[7.4.0.02,7]trideca-1(9),2(7),3,5,10,12-hexaen-8-one | |
---|---|---|
Source | PubChem | |
URL | https://pubchem.ncbi.nlm.nih.gov | |
Description | Data deposited in or computed by PubChem | |
InChI |
InChI=1S/C11H6N2O/c14-11-7-3-1-5-12-9(7)10-8(11)4-2-6-13-10/h1-6H | |
Source | PubChem | |
URL | https://pubchem.ncbi.nlm.nih.gov | |
Description | Data deposited in or computed by PubChem | |
InChI Key |
PFMTUGNLBQSHQC-UHFFFAOYSA-N | |
Source | PubChem | |
URL | https://pubchem.ncbi.nlm.nih.gov | |
Description | Data deposited in or computed by PubChem | |
Canonical SMILES |
C1=CC2=C(C3=C(C2=O)C=CC=N3)N=C1 | |
Source | PubChem | |
URL | https://pubchem.ncbi.nlm.nih.gov | |
Description | Data deposited in or computed by PubChem | |
Molecular Formula |
C11H6N2O | |
Source | PubChem | |
URL | https://pubchem.ncbi.nlm.nih.gov | |
Description | Data deposited in or computed by PubChem | |
DSSTOX Substance ID |
DTXSID10321556 | |
Record name | 4,5-Diazafluoren-9-one | |
Source | EPA DSSTox | |
URL | https://comptox.epa.gov/dashboard/DTXSID10321556 | |
Description | DSSTox provides a high quality public chemistry resource for supporting improved predictive toxicology. | |
Molecular Weight |
182.18 g/mol | |
Source | PubChem | |
URL | https://pubchem.ncbi.nlm.nih.gov | |
Description | Data deposited in or computed by PubChem | |
CAS No. |
50890-67-0 | |
Record name | 50890-67-0 | |
Source | DTP/NCI | |
URL | https://dtp.cancer.gov/dtpstandard/servlet/dwindex?searchtype=NSC&outputformat=html&searchlist=377648 | |
Description | The NCI Development Therapeutics Program (DTP) provides services and resources to the academic and private-sector research communities worldwide to facilitate the discovery and development of new cancer therapeutic agents. | |
Explanation | Unless otherwise indicated, all text within NCI products is free of copyright and may be reused without our permission. Credit the National Cancer Institute as the source. | |
Record name | 4,5-Diazafluoren-9-one | |
Source | EPA DSSTox | |
URL | https://comptox.epa.gov/dashboard/DTXSID10321556 | |
Description | DSSTox provides a high quality public chemistry resource for supporting improved predictive toxicology. | |
Record name | 4,5-Diazafluoren-9-one | |
Source | European Chemicals Agency (ECHA) | |
URL | https://echa.europa.eu/information-on-chemicals | |
Description | The European Chemicals Agency (ECHA) is an agency of the European Union which is the driving force among regulatory authorities in implementing the EU's groundbreaking chemicals legislation for the benefit of human health and the environment as well as for innovation and competitiveness. | |
Explanation | Use of the information, documents and data from the ECHA website is subject to the terms and conditions of this Legal Notice, and subject to other binding limitations provided for under applicable law, the information, documents and data made available on the ECHA website may be reproduced, distributed and/or used, totally or in part, for non-commercial purposes provided that ECHA is acknowledged as the source: "Source: European Chemicals Agency, http://echa.europa.eu/". Such acknowledgement must be included in each copy of the material. ECHA permits and encourages organisations and individuals to create links to the ECHA website under the following cumulative conditions: Links can only be made to webpages that provide a link to the Legal Notice page. | |
Synthesis routes and methods I
Procedure details
Synthesis routes and methods II
Procedure details
Retrosynthesis Analysis
AI-Powered Synthesis Planning: Our tool employs the Template_relevance Pistachio, Template_relevance Bkms_metabolic, Template_relevance Pistachio_ringbreaker, Template_relevance Reaxys, Template_relevance Reaxys_biocatalysis model, leveraging a vast database of chemical reactions to predict feasible synthetic routes.
One-Step Synthesis Focus: Specifically designed for one-step synthesis, it provides concise and direct routes for your target compounds, streamlining the synthesis process.
Accurate Predictions: Utilizing the extensive PISTACHIO, BKMS_METABOLIC, PISTACHIO_RINGBREAKER, REAXYS, REAXYS_BIOCATALYSIS database, our tool offers high-accuracy predictions, reflecting the latest in chemical research and data.
Strategy Settings
Precursor scoring | Relevance Heuristic |
---|---|
Min. plausibility | 0.01 |
Model | Template_relevance |
Template Set | Pistachio/Bkms_metabolic/Pistachio_ringbreaker/Reaxys/Reaxys_biocatalysis |
Top-N result to add to graph | 6 |
Feasible Synthetic Routes
A: 4,5-diazafluoren-9-one, often abbreviated as dafone, has a molecular formula of C11H6N2O and a molecular weight of 182.18 g/mol. []
ANone: this compound can be characterized using various spectroscopic techniques, including:
- NMR Spectroscopy (1H and 13C): Provides detailed information about the hydrogen and carbon environments within the molecule, confirming its structure and purity. [, , , , , ]
- IR Spectroscopy: Reveals characteristic absorption bands corresponding to specific functional groups, such as the carbonyl (C=O) stretch. [, , , ]
- UV-Vis Spectroscopy: Helps to analyze the electronic transitions within the molecule and determine its absorption and emission properties. [, , , , ]
- Mass Spectrometry (ESI-MS): Determines the molecular weight and fragmentation pattern of the compound, aiding in identification and characterization. [, ]
A: this compound often acts as a chelating ligand, coordinating to metal ions (e.g., Ag+, Cu2+, Zn2+, Pt2+, Ru2+) through its two nitrogen atoms. This chelation forms stable metal complexes with various geometries depending on the metal ion, its oxidation state, and the presence of other ligands. [, , , , , , , , , , , , , , , , , , , , ]
A: this compound itself can interact with DNA, but its metal complexes generally show stronger binding affinity. Studies suggest an intercalative mode of binding, where the planar aromatic structure of dafone inserts itself between DNA base pairs. [, , ]
A: this compound silver(I) nitrate complex (I) demonstrated broad-spectrum antibacterial activity against both Gram-positive and Gram-negative bacteria, including resistant clinical isolates. This activity was found to be comparable to or even better than some known antibiotics, especially against P. mirabilis, S. aureus, and K. pneumoniae. []
ANone: this compound and its metal complexes have shown potential in material science, particularly:
- Luminescent Materials: Europium tetracyanoplatinate incorporating this compound displays interesting luminescent properties, potentially useful in optoelectronic applications. []
- Hydrogel Loading: this compound silver(I) nitrate complex (I) can be loaded into hydrogels, allowing for controlled release and enhanced antibacterial activity. []
ANone: this compound has shown promise as a ligand in palladium-catalyzed reactions:
- Aerobic Dehydrogenative Heck Reaction: A Pd(OAc)2/4,5-diazafluoren-9-one catalyst system enables the aerobic dehydrogenative Heck alkenylation of ferrocene with alkenes, offering a more sustainable approach compared to traditional methods. []
- Aerobic Allylic C-H Acetoxylation: this compound acts as a crucial ligand in Pd(OAc)2-catalyzed aerobic allylic acetoxylation reactions. It supports catalytic turnover even without additional co-catalysts, unlike many other ligands. [, , ]
- Aerobic Acyloxylation of Allylic C-H Bonds: this compound, in conjunction with a Pd0 precatalyst and a quinone/iron phthalocyanine cocatalyst system, enables the aerobic acyloxylation of allylic C-H bonds with diverse carboxylic acids, expanding the scope of this reaction beyond acetic acid. []
- Aza-Wacker Reactions: this compound demonstrates "ligand-accelerated catalysis" in Pd(OAc)2-catalyzed oxidative cyclization of (E)-4-hexenyltosylamide, proving more effective than other bidentate ligands. []
A: Unlike many bidentate ligands that inhibit Pd-catalyzed aerobic oxidations, this compound promotes these reactions due to its ability to adopt both κ1- and κ2-coordination modes. This hemilabile character, confirmed by NMR and X-ray crystallography, allows for facile dissociation of one pyridyl ring, creating open coordination sites at the Pd center and facilitating key catalytic steps. [, ]
ANone: Density functional theory (DFT) calculations have been employed to:
- Investigate reaction mechanisms: DFT studies helped elucidate the reaction pathway of 3+2 cycloaddition reactions involving 9-oxo-4,5-diazafluorenium phenacylides, revealing a concerted nonsynchronous mechanism. []
- Understand ligand effects in catalysis: DFT calculations illuminated the influence of this compound and other chelating ligands on the energetics of the aza-Wacker reaction pathway, explaining their contrasting effects on catalytic activity. []
- Determine reduction potentials: DFT calculations complemented experimental data to determine the reduction potentials of (L)PdII(OAc)2 complexes, providing insights into how ligands like this compound modulate the oxidizing ability of PdII in catalytic reactions. []
ANone:
- Thermal Stability: Thermogravimetric analyses indicate that this compound-containing metal complexes generally exhibit good thermal stability. []
- Formulation Strategies: Encapsulation of this compound silver(I) nitrate complex (I) within hydrogels showcases a promising formulation strategy to enhance stability, control release, and improve its antibacterial efficacy. []
ANone: A variety of analytical techniques are employed, including:
- Liquid Chromatography-Mass Spectrometry (LC-MS): Enables the separation, identification, and quantification of this compound, offering high sensitivity and selectivity. []
- X-ray Diffraction: Provides detailed structural information for this compound and its metal complexes in the solid state, elucidating their coordination geometries and intermolecular interactions. [, , , , , , , , , , , , , , , , ]
- Elemental Analysis: Confirms the elemental composition of synthesized compounds, ensuring purity and consistency. [, ]
- Electrochemical Techniques (Cyclic Voltammetry): Provides insights into the redox properties of this compound and its metal complexes, which are relevant for understanding their reactivity and catalytic behavior. [, , , ]
Disclaimer and Information on In-Vitro Research Products
Please be aware that all articles and product information presented on BenchChem are intended solely for informational purposes. The products available for purchase on BenchChem are specifically designed for in-vitro studies, which are conducted outside of living organisms. In-vitro studies, derived from the Latin term "in glass," involve experiments performed in controlled laboratory settings using cells or tissues. It is important to note that these products are not categorized as medicines or drugs, and they have not received approval from the FDA for the prevention, treatment, or cure of any medical condition, ailment, or disease. We must emphasize that any form of bodily introduction of these products into humans or animals is strictly prohibited by law. It is essential to adhere to these guidelines to ensure compliance with legal and ethical standards in research and experimentation.