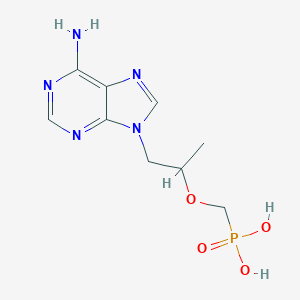
9-(2-Phosphonomethoxypropyl)adenine
Overview
Description
9-(2-Phosphonomethoxypropyl)adenine (PMPA), also known as (R)-PMPA or tenofovir, is an acyclic nucleoside phosphonate (ANP) first synthesized in 1987 by Holý and Rosenberg . Its structure features a phosphonomethoxypropyl side chain attached to the adenine base, enabling direct inhibition of viral polymerases without requiring initial phosphorylation . PMPA is clinically used as tenofovir disoproxil fumarate (TDF) or tenofovir alafenamide (TAF) for treating HIV-1 and chronic hepatitis B (HBV) infections due to its potent antiviral activity and favorable pharmacokinetic profile .
Preparation Methods
Synthetic Routes and Reaction Conditions
The synthesis of tenofovir-d6 involves the incorporation of deuterium atoms into the tenofovir molecule. One common method is the esterification of tenofovir with chloromethyl isopropyl carbonate in the presence of a base and a phase transfer catalyst . The reaction is typically carried out in a suitable solvent, followed by purification steps to obtain the deuterated product.
Industrial Production Methods
Industrial production of tenofovir-d6 follows similar synthetic routes but on a larger scale. The process involves stringent control of reaction conditions to ensure high yield and purity. The use of advanced purification techniques, such as high-performance liquid chromatography (HPLC), is common to achieve the desired quality .
Chemical Reactions Analysis
Types of Reactions
Tenofovir-d6 undergoes various chemical reactions, including:
Oxidation: Conversion to its oxidized form.
Reduction: Reduction of functional groups.
Substitution: Replacement of specific atoms or groups within the molecule.
Common Reagents and Conditions
Oxidation: Common oxidizing agents include hydrogen peroxide and potassium permanganate.
Reduction: Reducing agents such as sodium borohydride and lithium aluminum hydride are used.
Substitution: Reagents like halogens and nucleophiles are employed under controlled conditions.
Major Products Formed
The major products formed from these reactions depend on the specific reagents and conditions used. For example, oxidation may yield oxidized tenofovir-d6, while reduction can produce reduced forms of the compound .
Scientific Research Applications
Tenofovir-d6 is extensively used in scientific research, particularly in:
Chemistry: As an internal standard in analytical methods like HPLC and mass spectrometry for the quantification of tenofovir.
Biology: Studying the pharmacokinetics and metabolism of tenofovir in biological systems.
Medicine: Researching the efficacy and safety of tenofovir-based treatments for HIV and hepatitis B.
Industry: Quality control and validation of pharmaceutical formulations containing tenofovir
Mechanism of Action
Tenofovir-d6, like tenofovir, inhibits the activity of HIV-1 reverse transcriptase by competing with the natural substrate, deoxyadenosine 5’-triphosphate, and causing DNA chain termination. This prevents the replication of the virus. The molecular targets include the reverse transcriptase enzyme, and the pathways involved are those related to viral DNA synthesis .
Comparison with Similar Compounds
Comparative Analysis with Structural and Functional Analogues
Structural Analogues
PMEA (9-(2-Phosphonomethoxyethyl)adenine)
- Structural Difference : PMEA replaces PMPA’s propyl group with an ethyl chain.
- Activity : PMEA (adefovir) shows moderate anti-HIV activity but superior HBV inhibition. However, it exhibits higher nephrotoxicity than PMPA due to lower cellular uptake efficiency .
- Clinical Use: Marketed as adefovir dipivoxil for HBV, but largely replaced by tenofovir due to toxicity concerns .
HPMPA (9-(3-Hydroxy-2-phosphonomethoxypropyl)adenine)
- Structural Difference : Contains an additional hydroxyl group on the propyl chain.
- Activity : Broad-spectrum anti-DNA virus activity (e.g., cytomegalovirus, herpesviruses) but minimal antiretroviral effects. Unlike PMPA, HPMPA requires intracellular phosphorylation for activation .
- Limitations: Limited clinical utility due to poor oral bioavailability .
Functional Analogues
Cidofovir (HPMPC)
- Structural Difference : Cytosine replaces adenine in the HPMPA scaffold.
- Activity : Potent against dsDNA viruses (e.g., CMV, poxviruses) but inactive against retroviruses. Its mechanism involves competitive inhibition of viral DNA polymerases .
- Toxicity: Higher renal toxicity compared to PMPA, restricting its use to CMV retinitis in immunocompromised patients .
PMPDAP (9-(2-Phosphonomethoxypropyl)-2,6-diaminopurine)
- Structural Difference: 2,6-Diaminopurine replaces adenine.
- Activity : (R)-PMPDAP demonstrates 10-fold higher anti-HIV potency than PMPA in vitro but similar toxicity profiles. Its enhanced activity is attributed to stronger binding affinity to HIV reverse transcriptase (RT) .
Biochemical and Pharmacological Comparisons
Enzyme Inhibition Profiles
- Key Insight : PMPA’s diphosphate form (PMPApp) shows superior selectivity for viral RT over host DNA polymerases compared to PMEA and HPMPA .
Antiviral Efficacy and Toxicity
- Key Insight : PMPA’s high therapeutic index (>3000) underscores its clinical safety, while PMEA’s lower index reflects dose-limiting nephrotoxicity .
Resistance and Prodrug Strategies
Resistance Profiles
- PMPA : Rare HIV resistance mutations (e.g., K65R) occur with prolonged use, but less frequently than with AZT or lamivudine .
- PMEA : Rapid emergence of HBV resistance mutations (e.g., rtN236T) limits long-term efficacy .
Prodrug Innovations
- TDF (Tenofovir Disoproxil Fumarate): Enhances oral bioavailability by masking PMPA’s phosphonate group. However, high doses increase renal toxicity .
- TAF (Tenofovir Alafenamide): Newer prodrug with 90% lower plasma exposure than TDF, reducing off-target toxicity while maintaining antiviral efficacy .
Biological Activity
9-(2-Phosphonomethoxypropyl)adenine, commonly referred to as PMPA, is an acyclic nucleoside phosphonate analog of adenosine monophosphate (AMP). It has garnered significant attention due to its potent antiviral properties, particularly against retroviruses such as Human Immunodeficiency Virus (HIV) and Simian Immunodeficiency Virus (SIV). This article delves into the biological activity of PMPA, its mechanisms of action, pharmacokinetics, and relevant case studies.
PMPA functions primarily as an inhibitor of viral reverse transcriptase. Its mechanism involves the following steps:
- Phosphorylation : PMPA is phosphorylated within the cell to its active diphosphate form (PMPApp), which mimics natural nucleotides.
- Incorporation into Viral DNA : Once phosphorylated, PMPA competes with natural nucleotides for incorporation into the viral DNA during replication, leading to chain termination.
- Inhibition of Viral Replication : This incorporation effectively halts further viral replication, reducing viral load in infected cells.
Pharmacokinetics
PMPA exhibits remarkable stability against enzymatic degradation, particularly phosphorolytic cleavage by cellular esterases. This stability allows it to be taken up by cells in an unaltered form, enhancing its therapeutic efficacy. The compound's resistance to degradation contributes to a longer intracellular half-life for its active phosphorylated metabolites compared to other nucleoside analogs .
Biological Activity
PMPA has demonstrated significant antiviral activity against various viruses:
- HIV : PMPA has been shown to effectively inhibit HIV replication in vitro and in vivo. In laboratory studies, it significantly reduced plasma RNA levels of HIV and SIV .
- SIV : In a study involving cynomolgus macaques, PMPA treatment initiated shortly after SIV inoculation prevented the establishment of persistent infection even when therapy began 24 hours post-exposure .
Study 1: Efficacy Against SIV
In a controlled study with 24 cynomolgus macaques, different regimens of PMPA were tested for their ability to prevent persistent SIV infection. The results indicated that early initiation of PMPA treatment (within 24 hours post-inoculation) was crucial for preventing viral establishment. The study recorded various virologic outcomes, including plasma SIV RNA and PBMC-associated virus levels, demonstrating significant differences between treated and control groups (Table 1) .
Treatment Regimen | PBMC Viremia | Plasma SIV RNA | PCR Viral DNA |
---|---|---|---|
Control | 4/4 | 4/4 | 4/4 |
Treatment (24h) | 0/4 | 0/4 | 0/4 |
Treatment (48h) | 1/4 | 1/4 | 1/4 |
Treatment (72h) | 3/4 | 2/4 | 2/4 |
Study 2: Immunomodulatory Effects
Another study evaluated the immunomodulatory potential of PMPA. It was found that PMPA stimulated the secretion of various cytokines (IL-1β, IL-10) and chemokines (RANTES, MIP-1α) in mouse macrophages and lymphocytes. This immunomodulatory effect may enhance its antiviral efficacy by interfering with HIV replication and entry into cells .
Q & A
Q. Basic: What is the synthetic methodology for producing PMPA, and how can purity be validated?
Answer:
PMPA is synthesized via a multi-step process involving nucleophilic substitution and phosphorylation. Key intermediates include chloromethyl isopropyl carbonate (used in prodrug synthesis) and chiral resolution to isolate the (R)-enantiomer, which exhibits antiviral activity . Purity validation requires:
- HPLC with UV detection (λ = 260 nm) to quantify impurities (<1% single, <2% total) .
- NMR (¹H, ³¹P) to confirm phosphonate linkage and stereochemistry .
- Mass spectrometry (ESI-MS) to verify molecular weight (C₉H₁₄N₅O₄P; MW 287.21) .
Q. Basic: What is the mechanism of PMPA as an antiviral agent?
Answer:
PMPA is a nucleotide reverse transcriptase inhibitor (NtRTI). Its active diphosphate metabolite (PMPApp) competitively inhibits HIV-1 reverse transcriptase by mimicking dATP, terminating DNA chain elongation. Key steps include:
- Intracellular phosphorylation by cellular kinases to PMPApp .
- Binding affinity (Ki = 0.22 μM for HIV-1 RT) is 100-fold higher than tenofovir disoproxil fumarate (TDF) due to optimized phosphonate stability .
Q. Advanced: How do prodrug strategies (e.g., bis(POC)PMPA) enhance PMPA bioavailability, and what analytical methods track metabolic activation?
Answer:
Prodrugs like bis(isopropyloxymethylcarbonyl)PMPA mask the phosphonate’s negative charge, improving intestinal absorption. Post-absorption, esterases hydrolyze the prodrug to release PMPA . Methodologies include:
- Radiolabeled tracers (³H/¹⁴C-PMPA) to quantify prodrug conversion in plasma .
- LC-MS/MS to detect intermediate metabolites (e.g., mono-POC-PMPA) in hepatocyte assays .
- Caco-2 permeability assays showing bis(POC)PMPA has 10-fold higher absorption than PMPA .
Q. Advanced: How do structural modifications (e.g., N6-substitution) impact PMPA’s antiviral activity and resistance profile?
Answer:
Modifying the adenine N6 position alters binding to reverse transcriptase:
- N6-cyclopropyl derivatives reduce K65R mutant HIV resistance by enhancing hydrophobic interactions .
- SAR studies show N6-methylation decreases efficacy (EC₅₀ increases from 0.04 μM to >1 μM), while N6-ethyl retains potency . Assays include:
Q. Advanced: What experimental models resolve discrepancies between in vitro and in vivo PMPA efficacy data?
Answer:
In vitro assays (e.g., MT-4 cells) often overestimate potency due to artificial phosphorylation conditions. In vivo models address this via:
- Humanized BLT mice for HIV latency studies, showing PMPA reduces viral load by 2.5 log₁₀ vs. 4 log₁₀ in vitro .
- Rhesus macaque SHIV challenges to assess prophylactic efficacy (e.g., 90% protection at 10 mg/kg dosing) .
- PBMC assays with physiological nucleotide pools to mimic in vivo phosphorylation kinetics .
Q. Advanced: How is PMPA metabolism affected by cell-specific factors (e.g., lymphoid vs. renal tissue)?
Answer:
Metabolic activation varies by tissue:
- Lymphoid cells rapidly convert PMPA to PMPApp via adenylate kinase 2 (AK2), achieving intracellular [PMPApp] of 15 μM .
- Renal proximal tubules lack efficient phosphorylation, leading to accumulation of unmetabolized PMPA and potential nephrotoxicity. Methods to study this:
- Cellular fractionation + HPLC to quantify PMPA/PMPApp ratios .
- CRISPR-KO models of AK2 to validate phosphorylation dependency .
Q. Advanced: What combinatorial approaches optimize PMPA’s synergy with other antiretrovirals?
Answer:
PMPA synergizes with NRTIs (e.g., zidovudine) and integrase inhibitors (e.g., dolutegravir):
- Chou-Talalay assays show combination indices (CI) <0.5 for PMPA + zidovudine in PBMCs .
- Mechanistic synergy arises from PMPA’s chain termination and zidovudine’s competitive inhibition.
- In vivo validation in humanized mice demonstrates 50% lower PMPA dose requirements in combinational regimens .
Q. Advanced: How do phosphonate analogs (e.g., PMPA vs. PMEA) differ in antiviral spectrum and toxicity?
Answer:
- PMPA (EC₅₀ = 0.04 μM for HIV-1) has higher specificity than PMEA (EC₅₀ = 1.2 μM) due to its propyl side chain reducing off-target binding .
- Toxicity profiles : PMPA’s nephrotoxicity (IC₅₀ = 450 μM in renal cells) is milder than PMEA’s hematologic toxicity (IC₅₀ = 50 μM) .
- Assays : Comparative cytotoxicity screens in HEK-293 and CEM-T cells .
Q. Advanced: What computational methods predict PMPA resistance mutations?
Answer:
- Molecular dynamics (MD) simulations of HIV RT-PMPApp complexes identify residues (K65, K70) prone to mutation .
- Machine learning models trained on clinical isolate genotypic data predict K65R emergence with 89% accuracy .
- Free energy perturbation (FEP) calculates ΔΔG for mutant RT-PMPApp binding, correlating with phenotypic resistance .
Q. Advanced: How can PMPA’s pharmacokinetics be modeled to optimize dosing regimens?
Answer:
- Physiologically based pharmacokinetic (PBPK) models integrate:
- Non-compartmental analysis (NCA) of plasma concentration-time curves to calculate t₁/₂ (17 hr) and AUC₀–24 (3.2 μg·hr/mL) .
Properties
IUPAC Name |
1-(6-aminopurin-9-yl)propan-2-yloxymethylphosphonic acid | |
---|---|---|
Source | PubChem | |
URL | https://pubchem.ncbi.nlm.nih.gov | |
Description | Data deposited in or computed by PubChem | |
InChI |
InChI=1S/C9H14N5O4P/c1-6(18-5-19(15,16)17)2-14-4-13-7-8(10)11-3-12-9(7)14/h3-4,6H,2,5H2,1H3,(H2,10,11,12)(H2,15,16,17) | |
Source | PubChem | |
URL | https://pubchem.ncbi.nlm.nih.gov | |
Description | Data deposited in or computed by PubChem | |
InChI Key |
SGOIRFVFHAKUTI-UHFFFAOYSA-N | |
Source | PubChem | |
URL | https://pubchem.ncbi.nlm.nih.gov | |
Description | Data deposited in or computed by PubChem | |
Canonical SMILES |
CC(CN1C=NC2=C(N=CN=C21)N)OCP(=O)(O)O | |
Source | PubChem | |
URL | https://pubchem.ncbi.nlm.nih.gov | |
Description | Data deposited in or computed by PubChem | |
Molecular Formula |
C9H14N5O4P | |
Source | PubChem | |
URL | https://pubchem.ncbi.nlm.nih.gov | |
Description | Data deposited in or computed by PubChem | |
DSSTOX Substance ID |
DTXSID30861388 | |
Record name | ({[1-(6-Amino-9H-purin-9-yl)propan-2-yl]oxy}methyl)phosphonic acid | |
Source | EPA DSSTox | |
URL | https://comptox.epa.gov/dashboard/DTXSID30861388 | |
Description | DSSTox provides a high quality public chemistry resource for supporting improved predictive toxicology. | |
Molecular Weight |
287.21 g/mol | |
Source | PubChem | |
URL | https://pubchem.ncbi.nlm.nih.gov | |
Description | Data deposited in or computed by PubChem | |
CAS No. |
107021-12-5 | |
Record name | Tenofovir (parent) | |
Source | ChemIDplus | |
URL | https://pubchem.ncbi.nlm.nih.gov/substance/?source=chemidplus&sourceid=0107021125 | |
Description | ChemIDplus is a free, web search system that provides access to the structure and nomenclature authority files used for the identification of chemical substances cited in National Library of Medicine (NLM) databases, including the TOXNET system. | |
Record name | ({[1-(6-Amino-9H-purin-9-yl)propan-2-yl]oxy}methyl)phosphonic acid | |
Source | EPA DSSTox | |
URL | https://comptox.epa.gov/dashboard/DTXSID30861388 | |
Description | DSSTox provides a high quality public chemistry resource for supporting improved predictive toxicology. | |
Retrosynthesis Analysis
AI-Powered Synthesis Planning: Our tool employs the Template_relevance Pistachio, Template_relevance Bkms_metabolic, Template_relevance Pistachio_ringbreaker, Template_relevance Reaxys, Template_relevance Reaxys_biocatalysis model, leveraging a vast database of chemical reactions to predict feasible synthetic routes.
One-Step Synthesis Focus: Specifically designed for one-step synthesis, it provides concise and direct routes for your target compounds, streamlining the synthesis process.
Accurate Predictions: Utilizing the extensive PISTACHIO, BKMS_METABOLIC, PISTACHIO_RINGBREAKER, REAXYS, REAXYS_BIOCATALYSIS database, our tool offers high-accuracy predictions, reflecting the latest in chemical research and data.
Strategy Settings
Precursor scoring | Relevance Heuristic |
---|---|
Min. plausibility | 0.01 |
Model | Template_relevance |
Template Set | Pistachio/Bkms_metabolic/Pistachio_ringbreaker/Reaxys/Reaxys_biocatalysis |
Top-N result to add to graph | 6 |
Feasible Synthetic Routes
Disclaimer and Information on In-Vitro Research Products
Please be aware that all articles and product information presented on BenchChem are intended solely for informational purposes. The products available for purchase on BenchChem are specifically designed for in-vitro studies, which are conducted outside of living organisms. In-vitro studies, derived from the Latin term "in glass," involve experiments performed in controlled laboratory settings using cells or tissues. It is important to note that these products are not categorized as medicines or drugs, and they have not received approval from the FDA for the prevention, treatment, or cure of any medical condition, ailment, or disease. We must emphasize that any form of bodily introduction of these products into humans or animals is strictly prohibited by law. It is essential to adhere to these guidelines to ensure compliance with legal and ethical standards in research and experimentation.