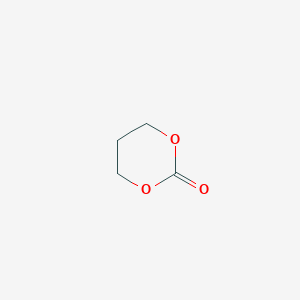
1,3-Dioxan-2-one
Overview
Description
1,3-Dioxan-2-one is a six-membered cyclic carbonate ester with the chemical formula C4H6O3.
Preparation Methods
Synthetic Routes and Reaction Conditions
1,3-Dioxan-2-one can be synthesized through various methods. One common approach involves the reaction of 1,3-diols with phosgene derivatives or carbon monoxide . Another method utilizes the carboxylative cyclization of 1,3-diols in the presence of carbon dioxide and catalysts such as cerium oxide and 2-cyanopyridine . Additionally, photo-aerobic selenium-π-acid multicatalysis has been employed to synthesize 1,3-dioxan-2-ones from homoallylic carbonic acid esters .
Industrial Production Methods
Industrial production of this compound often involves the use of carbon dioxide as a C1 source, leveraging its availability and cost-effectiveness. The process typically includes the use of catalysts and specific reaction conditions to ensure high yield and purity of the product .
Chemical Reactions Analysis
Types of Reactions
1,3-Dioxan-2-one undergoes various chemical reactions, including:
Oxidation: The compound can be oxidized under specific conditions to form different products.
Reduction: Reduction reactions can yield tertiary alcohols and other derivatives.
Substitution: Substitution reactions involving this compound can lead to the formation of various substituted cyclic carbonates.
Common Reagents and Conditions
Common reagents used in these reactions include strong bases, carbon dioxide, cerium oxide, and 2-cyanopyridine . Reaction conditions often involve specific temperatures, pressures, and catalysts to achieve the desired transformations .
Major Products
Major products formed from these reactions include aliphatic polycarbonates and polyurethanes, which are of significant interest in material sciences and industrial applications .
Scientific Research Applications
1,3-Dioxan-2-one has a wide range of applications in scientific research:
Mechanism of Action
The mechanism of action of 1,3-dioxan-2-one involves its ability to undergo ring-opening polymerization, which is a key process in the formation of polycarbonates and polyurethanes . The compound’s molecular structure allows it to interact with catalysts and reagents, facilitating the formation of high-molecular-weight polymers .
Comparison with Similar Compounds
Similar Compounds
1,3-Dioxolan-2-one: Another cyclic carbonate ester with similar properties and applications.
1,4-Dioxane: A six-membered cyclic ether with different chemical properties and uses.
1,3-Dioxane: A cyclic ether used as a solvent and in various chemical reactions.
Uniqueness
1,3-Dioxan-2-one is unique due to its ability to form high-performance polymers through ring-opening polymerization. Its versatility in various chemical reactions and applications in material sciences and industrial processes sets it apart from other similar compounds .
Biological Activity
1,3-Dioxan-2-one, also known as trimethylene carbonate (TMC), is a six-membered cyclic carbonate with significant relevance in the field of polymer chemistry, particularly for its applications in biomedical materials due to its favorable biological properties. This article explores the biological activity of this compound, focusing on its synthesis, polymerization, biocompatibility, and potential applications in medical devices and drug delivery systems.
This compound has a molecular formula of and appears as a colorless solid with a melting point of approximately 45.0 to 49.0 °C. It is soluble in methanol and typically stored under inert gas conditions to prevent degradation. The most common methods for synthesizing this compound include:
- Reaction with Phosgene or Carbon Dioxide : Utilizing 1,3-diols as starting materials.
- Catalytic Methods : Recent advancements have highlighted the use of carbon dioxide as a sustainable carbon source in synthesis, employing catalysts like 1,8-diazabicyclo[5.4.0]undec-7-ene to enhance yields under mild conditions .
Biocompatibility and Toxicity
The biological activity of this compound is characterized by its low toxicity and biodegradability , making it a suitable candidate for various biomedical applications. Studies have shown that polymers derived from TMC exhibit excellent biocompatibility, which is crucial for medical applications such as:
- Sutures
- Drug Delivery Systems
- Tissue Engineering Scaffolds
These materials are designed to degrade naturally within the body, minimizing long-term environmental impact while providing mechanical support during healing processes .
Polymerization Characteristics
This compound can undergo ring-opening polymerization (ROP), leading to the formation of high molecular weight poly(trimethylene carbonate). This polymerization can be initiated using both cationic and anionic initiators. The resulting polymers are of interest for their mechanical properties and biodegradability, which are critical in medical applications .
Study on PPAR Activation
A study evaluated the effects of dioxane derivatives on human PPAR (peroxisome proliferator-activated receptor) transactivation activity. Although this study primarily focused on related compounds, it provides insights into the potential metabolic effects of dioxane derivatives like this compound. The findings indicated that certain modifications could enhance biological activity relevant to metabolic disorders .
Biodegradable Polymers
Research has demonstrated that polymers synthesized from this compound exhibit good mechanical properties suitable for various medical applications. For instance:
Property | Value |
---|---|
Biodegradation Time | Varies (weeks to months) |
Mechanical Strength | Comparable to PLA |
Cytotoxicity | Low (biocompatible) |
This table summarizes key properties that highlight the suitability of TMC-derived polymers for biomedical applications.
Properties
IUPAC Name |
1,3-dioxan-2-one | |
---|---|---|
Source | PubChem | |
URL | https://pubchem.ncbi.nlm.nih.gov | |
Description | Data deposited in or computed by PubChem | |
InChI |
InChI=1S/C4H6O3/c5-4-6-2-1-3-7-4/h1-3H2 | |
Source | PubChem | |
URL | https://pubchem.ncbi.nlm.nih.gov | |
Description | Data deposited in or computed by PubChem | |
InChI Key |
YFHICDDUDORKJB-UHFFFAOYSA-N | |
Source | PubChem | |
URL | https://pubchem.ncbi.nlm.nih.gov | |
Description | Data deposited in or computed by PubChem | |
Canonical SMILES |
C1COC(=O)OC1 | |
Source | PubChem | |
URL | https://pubchem.ncbi.nlm.nih.gov | |
Description | Data deposited in or computed by PubChem | |
Molecular Formula |
C4H6O3 | |
Source | PubChem | |
URL | https://pubchem.ncbi.nlm.nih.gov | |
Description | Data deposited in or computed by PubChem | |
Related CAS |
31852-84-3 | |
Record name | Trimethylene carbonate homopolymer | |
Source | CAS Common Chemistry | |
URL | https://commonchemistry.cas.org/detail?cas_rn=31852-84-3 | |
Description | CAS Common Chemistry is an open community resource for accessing chemical information. Nearly 500,000 chemical substances from CAS REGISTRY cover areas of community interest, including common and frequently regulated chemicals, and those relevant to high school and undergraduate chemistry classes. This chemical information, curated by our expert scientists, is provided in alignment with our mission as a division of the American Chemical Society. | |
Explanation | The data from CAS Common Chemistry is provided under a CC-BY-NC 4.0 license, unless otherwise stated. | |
DSSTOX Substance ID |
DTXSID20953766 | |
Record name | Trimethylene carbonate | |
Source | EPA DSSTox | |
URL | https://comptox.epa.gov/dashboard/DTXSID20953766 | |
Description | DSSTox provides a high quality public chemistry resource for supporting improved predictive toxicology. | |
Molecular Weight |
102.09 g/mol | |
Source | PubChem | |
URL | https://pubchem.ncbi.nlm.nih.gov | |
Description | Data deposited in or computed by PubChem | |
CAS No. |
2453-03-4 | |
Record name | Trimethylene carbonate | |
Source | CAS Common Chemistry | |
URL | https://commonchemistry.cas.org/detail?cas_rn=2453-03-4 | |
Description | CAS Common Chemistry is an open community resource for accessing chemical information. Nearly 500,000 chemical substances from CAS REGISTRY cover areas of community interest, including common and frequently regulated chemicals, and those relevant to high school and undergraduate chemistry classes. This chemical information, curated by our expert scientists, is provided in alignment with our mission as a division of the American Chemical Society. | |
Explanation | The data from CAS Common Chemistry is provided under a CC-BY-NC 4.0 license, unless otherwise stated. | |
Record name | Trimethylene carbonate | |
Source | ChemIDplus | |
URL | https://pubchem.ncbi.nlm.nih.gov/substance/?source=chemidplus&sourceid=0002453034 | |
Description | ChemIDplus is a free, web search system that provides access to the structure and nomenclature authority files used for the identification of chemical substances cited in National Library of Medicine (NLM) databases, including the TOXNET system. | |
Record name | Trimethylene carbonate | |
Source | EPA DSSTox | |
URL | https://comptox.epa.gov/dashboard/DTXSID20953766 | |
Description | DSSTox provides a high quality public chemistry resource for supporting improved predictive toxicology. | |
Record name | 1,3-Dioxan-2-one | |
Source | European Chemicals Agency (ECHA) | |
URL | https://echa.europa.eu/substance-information/-/substanceinfo/100.114.239 | |
Description | The European Chemicals Agency (ECHA) is an agency of the European Union which is the driving force among regulatory authorities in implementing the EU's groundbreaking chemicals legislation for the benefit of human health and the environment as well as for innovation and competitiveness. | |
Explanation | Use of the information, documents and data from the ECHA website is subject to the terms and conditions of this Legal Notice, and subject to other binding limitations provided for under applicable law, the information, documents and data made available on the ECHA website may be reproduced, distributed and/or used, totally or in part, for non-commercial purposes provided that ECHA is acknowledged as the source: "Source: European Chemicals Agency, http://echa.europa.eu/". Such acknowledgement must be included in each copy of the material. ECHA permits and encourages organisations and individuals to create links to the ECHA website under the following cumulative conditions: Links can only be made to webpages that provide a link to the Legal Notice page. | |
Record name | TRIMETHYLENE CARBONATE | |
Source | FDA Global Substance Registration System (GSRS) | |
URL | https://gsrs.ncats.nih.gov/ginas/app/beta/substances/4316AQ174Q | |
Description | The FDA Global Substance Registration System (GSRS) enables the efficient and accurate exchange of information on what substances are in regulated products. Instead of relying on names, which vary across regulatory domains, countries, and regions, the GSRS knowledge base makes it possible for substances to be defined by standardized, scientific descriptions. | |
Explanation | Unless otherwise noted, the contents of the FDA website (www.fda.gov), both text and graphics, are not copyrighted. They are in the public domain and may be republished, reprinted and otherwise used freely by anyone without the need to obtain permission from FDA. Credit to the U.S. Food and Drug Administration as the source is appreciated but not required. | |
Synthesis routes and methods
Procedure details
Retrosynthesis Analysis
AI-Powered Synthesis Planning: Our tool employs the Template_relevance Pistachio, Template_relevance Bkms_metabolic, Template_relevance Pistachio_ringbreaker, Template_relevance Reaxys, Template_relevance Reaxys_biocatalysis model, leveraging a vast database of chemical reactions to predict feasible synthetic routes.
One-Step Synthesis Focus: Specifically designed for one-step synthesis, it provides concise and direct routes for your target compounds, streamlining the synthesis process.
Accurate Predictions: Utilizing the extensive PISTACHIO, BKMS_METABOLIC, PISTACHIO_RINGBREAKER, REAXYS, REAXYS_BIOCATALYSIS database, our tool offers high-accuracy predictions, reflecting the latest in chemical research and data.
Strategy Settings
Precursor scoring | Relevance Heuristic |
---|---|
Min. plausibility | 0.01 |
Model | Template_relevance |
Template Set | Pistachio/Bkms_metabolic/Pistachio_ringbreaker/Reaxys/Reaxys_biocatalysis |
Top-N result to add to graph | 6 |
Feasible Synthetic Routes
Disclaimer and Information on In-Vitro Research Products
Please be aware that all articles and product information presented on BenchChem are intended solely for informational purposes. The products available for purchase on BenchChem are specifically designed for in-vitro studies, which are conducted outside of living organisms. In-vitro studies, derived from the Latin term "in glass," involve experiments performed in controlled laboratory settings using cells or tissues. It is important to note that these products are not categorized as medicines or drugs, and they have not received approval from the FDA for the prevention, treatment, or cure of any medical condition, ailment, or disease. We must emphasize that any form of bodily introduction of these products into humans or animals is strictly prohibited by law. It is essential to adhere to these guidelines to ensure compliance with legal and ethical standards in research and experimentation.