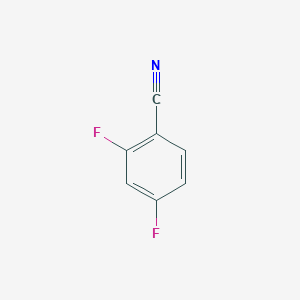
2,4-Difluorobenzonitrile
Overview
Description
2,4-Difluorobenzonitrile is an organic compound with the molecular formula C7H3F2N. It is a white crystalline solid that is primarily used as an intermediate in the synthesis of various chemical products. The compound is characterized by the presence of two fluorine atoms attached to the benzene ring at the 2 and 4 positions, along with a nitrile group (-CN) at the 1 position.
Preparation Methods
Synthetic Routes and Reaction Conditions: 2,4-Difluorobenzonitrile can be synthesized through several methods. One common method involves the reaction of 2,4-difluorobromobenzene with sodium cyanide in the presence of a catalyst such as cuprous iodide and potassium iodide. The reaction is typically carried out in a solvent like toluene under nitrogen protection at a temperature of around 100°C for 48 hours . Another method involves the use of 2,4-difluorobenzoic acid, which is first converted to 2,4-difluorobenzoyl chloride using thionyl chloride, followed by reaction with ammonia to produce 2,4-difluorobenzamide, which is then dehydrated to form this compound .
Industrial Production Methods: The industrial production of this compound often involves the use of continuous flow reactors to ensure consistent quality and yield. The process typically includes the use of phase transfer catalysts and recycling of solvents and catalysts to minimize waste and reduce production costs .
Chemical Reactions Analysis
Types of Reactions: 2,4-Difluorobenzonitrile undergoes various chemical reactions, including:
Substitution Reactions: The fluorine atoms can be substituted with other functional groups using appropriate reagents.
Reduction Reactions: The nitrile group can be reduced to an amine using reducing agents like lithium aluminum hydride.
Oxidation Reactions: The compound can undergo oxidation to form corresponding acids or other derivatives.
Common Reagents and Conditions:
Substitution: Reagents like sodium methoxide or potassium tert-butoxide in polar aprotic solvents.
Reduction: Lithium aluminum hydride in dry ether.
Oxidation: Potassium permanganate in acidic or basic medium.
Major Products:
Substitution: Products like 2,4-difluoroaniline.
Reduction: 2,4-difluorobenzylamine.
Oxidation: 2,4-difluorobenzoic acid.
Scientific Research Applications
2,4-Difluorobenzonitrile is widely used in scientific research and industrial applications:
Biology: Used in the study of enzyme inhibitors and receptor binding studies.
Medicine: As a precursor in the synthesis of drugs and therapeutic agents.
Industry: In the production of polymers and specialty chemicals.
Mechanism of Action
The mechanism of action of 2,4-difluorobenzonitrile depends on its application. In chemical reactions, it acts as a nucleophile or electrophile depending on the reaction conditions. In biological systems, it may interact with specific enzymes or receptors, inhibiting their activity or altering their function. The exact molecular targets and pathways involved vary based on the specific application and the structure of the derivatives formed .
Comparison with Similar Compounds
- 2,6-Difluorobenzonitrile
- 2,4-Dichlorobenzonitrile
- 2,3-Difluorobenzonitrile
- 4-Fluorobenzonitrile
Comparison: 2,4-Difluorobenzonitrile is unique due to the specific positioning of the fluorine atoms, which influences its reactivity and the types of reactions it can undergo. Compared to 2,6-difluorobenzonitrile, it has different electronic and steric properties, making it suitable for different synthetic applications. The presence of two fluorine atoms also enhances its stability and resistance to metabolic degradation, making it a valuable intermediate in pharmaceutical synthesis .
Properties
IUPAC Name |
2,4-difluorobenzonitrile | |
---|---|---|
Source | PubChem | |
URL | https://pubchem.ncbi.nlm.nih.gov | |
Description | Data deposited in or computed by PubChem | |
InChI |
InChI=1S/C7H3F2N/c8-6-2-1-5(4-10)7(9)3-6/h1-3H | |
Source | PubChem | |
URL | https://pubchem.ncbi.nlm.nih.gov | |
Description | Data deposited in or computed by PubChem | |
InChI Key |
LJFDXXUKKMEQKE-UHFFFAOYSA-N | |
Source | PubChem | |
URL | https://pubchem.ncbi.nlm.nih.gov | |
Description | Data deposited in or computed by PubChem | |
Canonical SMILES |
C1=CC(=C(C=C1F)F)C#N | |
Source | PubChem | |
URL | https://pubchem.ncbi.nlm.nih.gov | |
Description | Data deposited in or computed by PubChem | |
Molecular Formula |
C7H3F2N | |
Source | PubChem | |
URL | https://pubchem.ncbi.nlm.nih.gov | |
Description | Data deposited in or computed by PubChem | |
DSSTOX Substance ID |
DTXSID70192582 | |
Record name | 2,4-Difluorobenzonitrile | |
Source | EPA DSSTox | |
URL | https://comptox.epa.gov/dashboard/DTXSID70192582 | |
Description | DSSTox provides a high quality public chemistry resource for supporting improved predictive toxicology. | |
Molecular Weight |
139.10 g/mol | |
Source | PubChem | |
URL | https://pubchem.ncbi.nlm.nih.gov | |
Description | Data deposited in or computed by PubChem | |
CAS No. |
3939-09-1 | |
Record name | 2,4-Difluorobenzonitrile | |
Source | CAS Common Chemistry | |
URL | https://commonchemistry.cas.org/detail?cas_rn=3939-09-1 | |
Description | CAS Common Chemistry is an open community resource for accessing chemical information. Nearly 500,000 chemical substances from CAS REGISTRY cover areas of community interest, including common and frequently regulated chemicals, and those relevant to high school and undergraduate chemistry classes. This chemical information, curated by our expert scientists, is provided in alignment with our mission as a division of the American Chemical Society. | |
Explanation | The data from CAS Common Chemistry is provided under a CC-BY-NC 4.0 license, unless otherwise stated. | |
Record name | 2,4-Difluorobenzonitrile | |
Source | ChemIDplus | |
URL | https://pubchem.ncbi.nlm.nih.gov/substance/?source=chemidplus&sourceid=0003939091 | |
Description | ChemIDplus is a free, web search system that provides access to the structure and nomenclature authority files used for the identification of chemical substances cited in National Library of Medicine (NLM) databases, including the TOXNET system. | |
Record name | 2,4-Difluorobenzonitrile | |
Source | EPA DSSTox | |
URL | https://comptox.epa.gov/dashboard/DTXSID70192582 | |
Description | DSSTox provides a high quality public chemistry resource for supporting improved predictive toxicology. | |
Record name | 2,4-difluorobenzonitrile | |
Source | European Chemicals Agency (ECHA) | |
URL | https://echa.europa.eu/substance-information/-/substanceinfo/100.021.386 | |
Description | The European Chemicals Agency (ECHA) is an agency of the European Union which is the driving force among regulatory authorities in implementing the EU's groundbreaking chemicals legislation for the benefit of human health and the environment as well as for innovation and competitiveness. | |
Explanation | Use of the information, documents and data from the ECHA website is subject to the terms and conditions of this Legal Notice, and subject to other binding limitations provided for under applicable law, the information, documents and data made available on the ECHA website may be reproduced, distributed and/or used, totally or in part, for non-commercial purposes provided that ECHA is acknowledged as the source: "Source: European Chemicals Agency, http://echa.europa.eu/". Such acknowledgement must be included in each copy of the material. ECHA permits and encourages organisations and individuals to create links to the ECHA website under the following cumulative conditions: Links can only be made to webpages that provide a link to the Legal Notice page. | |
Record name | 2,4-DIFLUOROBENZONITRILE | |
Source | FDA Global Substance Registration System (GSRS) | |
URL | https://gsrs.ncats.nih.gov/ginas/app/beta/substances/T4A8BQ9ZWK | |
Description | The FDA Global Substance Registration System (GSRS) enables the efficient and accurate exchange of information on what substances are in regulated products. Instead of relying on names, which vary across regulatory domains, countries, and regions, the GSRS knowledge base makes it possible for substances to be defined by standardized, scientific descriptions. | |
Explanation | Unless otherwise noted, the contents of the FDA website (www.fda.gov), both text and graphics, are not copyrighted. They are in the public domain and may be republished, reprinted and otherwise used freely by anyone without the need to obtain permission from FDA. Credit to the U.S. Food and Drug Administration as the source is appreciated but not required. | |
Retrosynthesis Analysis
AI-Powered Synthesis Planning: Our tool employs the Template_relevance Pistachio, Template_relevance Bkms_metabolic, Template_relevance Pistachio_ringbreaker, Template_relevance Reaxys, Template_relevance Reaxys_biocatalysis model, leveraging a vast database of chemical reactions to predict feasible synthetic routes.
One-Step Synthesis Focus: Specifically designed for one-step synthesis, it provides concise and direct routes for your target compounds, streamlining the synthesis process.
Accurate Predictions: Utilizing the extensive PISTACHIO, BKMS_METABOLIC, PISTACHIO_RINGBREAKER, REAXYS, REAXYS_BIOCATALYSIS database, our tool offers high-accuracy predictions, reflecting the latest in chemical research and data.
Strategy Settings
Precursor scoring | Relevance Heuristic |
---|---|
Min. plausibility | 0.01 |
Model | Template_relevance |
Template Set | Pistachio/Bkms_metabolic/Pistachio_ringbreaker/Reaxys/Reaxys_biocatalysis |
Top-N result to add to graph | 6 |
Feasible Synthetic Routes
Disclaimer and Information on In-Vitro Research Products
Please be aware that all articles and product information presented on BenchChem are intended solely for informational purposes. The products available for purchase on BenchChem are specifically designed for in-vitro studies, which are conducted outside of living organisms. In-vitro studies, derived from the Latin term "in glass," involve experiments performed in controlled laboratory settings using cells or tissues. It is important to note that these products are not categorized as medicines or drugs, and they have not received approval from the FDA for the prevention, treatment, or cure of any medical condition, ailment, or disease. We must emphasize that any form of bodily introduction of these products into humans or animals is strictly prohibited by law. It is essential to adhere to these guidelines to ensure compliance with legal and ethical standards in research and experimentation.