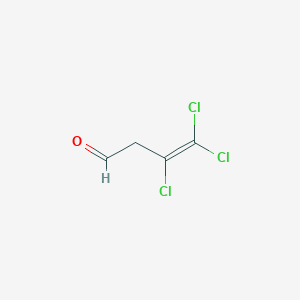
3,4,4-Trichloro-3-butenal
Overview
Description
3,4,4-Trichloro-3-butenal is an organic compound with the molecular formula C4H3Cl3O
Preparation Methods
Synthetic Routes and Reaction Conditions
3,4,4-Trichloro-3-butenal can be synthesized through the chlorination of butenal. The reaction typically involves the use of chlorine gas in the presence of a catalyst such as iron(III) chloride. The reaction conditions include maintaining a temperature range of 0-5°C to control the exothermic nature of the chlorination process.
Industrial Production Methods
In an industrial setting, the production of this compound involves a continuous flow process where butenal is fed into a reactor along with chlorine gas. The reaction is carried out under controlled temperature and pressure conditions to ensure high yield and purity of the product. The crude product is then purified through distillation or recrystallization.
Chemical Reactions Analysis
Types of Reactions
3,4,4-Trichloro-3-butenal undergoes various types of chemical reactions, including:
Oxidation: It can be oxidized to form 3,4,4-trichloro-3-butenoic acid.
Reduction: Reduction of this compound can yield 3,4,4-trichlorobutanol.
Substitution: The compound can undergo nucleophilic substitution reactions where the chlorine atoms are replaced by other nucleophiles.
Common Reagents and Conditions
Oxidation: Common oxidizing agents include potassium permanganate and chromium trioxide.
Reduction: Reducing agents such as lithium aluminum hydride or sodium borohydride are used.
Substitution: Nucleophiles like amines, alcohols, or thiols can be used under basic conditions.
Major Products Formed
Oxidation: 3,4,4-trichloro-3-butenoic acid.
Reduction: 3,4,4-trichlorobutanol.
Substitution: Various substituted derivatives depending on the nucleophile used.
Scientific Research Applications
Chemistry: It is used as an intermediate in the synthesis of other chlorinated compounds.
Biology: Research has explored its effects on biological systems, particularly its potential as a biocide.
Medicine: Studies have investigated its potential use in the development of pharmaceuticals, particularly as a precursor for active pharmaceutical ingredients.
Industry: It is used in the production of agrochemicals and other specialty chemicals.
Mechanism of Action
The mechanism by which 3,4,4-Trichloro-3-butenal exerts its effects involves its reactivity with nucleophiles. The compound can form covalent bonds with nucleophilic sites in biological molecules, leading to potential biocidal activity. The molecular targets include enzymes and other proteins with nucleophilic functional groups.
Comparison with Similar Compounds
Similar Compounds
- 3,4,4-Trichloro-3-butenoic acid
- 3,4,4-Trichlorobutanol
- 3,4,4-Trichloro-3-butenoates
Uniqueness
3,4,4-Trichloro-3-butenal is unique due to its highly reactive aldehyde group combined with multiple chlorine atoms. This combination makes it a versatile intermediate for various chemical reactions and applications. Its reactivity and potential for forming multiple derivatives distinguish it from other similar compounds.
Properties
IUPAC Name |
3,4,4-trichlorobut-3-enal | |
---|---|---|
Source | PubChem | |
URL | https://pubchem.ncbi.nlm.nih.gov | |
Description | Data deposited in or computed by PubChem | |
InChI |
InChI=1S/C4H3Cl3O/c5-3(1-2-8)4(6)7/h2H,1H2 | |
Source | PubChem | |
URL | https://pubchem.ncbi.nlm.nih.gov | |
Description | Data deposited in or computed by PubChem | |
InChI Key |
MOOVCLOCRWBFIZ-UHFFFAOYSA-N | |
Source | PubChem | |
URL | https://pubchem.ncbi.nlm.nih.gov | |
Description | Data deposited in or computed by PubChem | |
Canonical SMILES |
C(C=O)C(=C(Cl)Cl)Cl | |
Source | PubChem | |
URL | https://pubchem.ncbi.nlm.nih.gov | |
Description | Data deposited in or computed by PubChem | |
Molecular Formula |
C4H3Cl3O | |
Source | PubChem | |
URL | https://pubchem.ncbi.nlm.nih.gov | |
Description | Data deposited in or computed by PubChem | |
DSSTOX Substance ID |
DTXSID20338933 | |
Record name | 3,4,4-Trichloro-3-butenal | |
Source | EPA DSSTox | |
URL | https://comptox.epa.gov/dashboard/DTXSID20338933 | |
Description | DSSTox provides a high quality public chemistry resource for supporting improved predictive toxicology. | |
Molecular Weight |
173.42 g/mol | |
Source | PubChem | |
URL | https://pubchem.ncbi.nlm.nih.gov | |
Description | Data deposited in or computed by PubChem | |
CAS No. |
108562-62-5 | |
Record name | 3,4,4-Trichloro-3-butenal | |
Source | EPA DSSTox | |
URL | https://comptox.epa.gov/dashboard/DTXSID20338933 | |
Description | DSSTox provides a high quality public chemistry resource for supporting improved predictive toxicology. | |
Retrosynthesis Analysis
AI-Powered Synthesis Planning: Our tool employs the Template_relevance Pistachio, Template_relevance Bkms_metabolic, Template_relevance Pistachio_ringbreaker, Template_relevance Reaxys, Template_relevance Reaxys_biocatalysis model, leveraging a vast database of chemical reactions to predict feasible synthetic routes.
One-Step Synthesis Focus: Specifically designed for one-step synthesis, it provides concise and direct routes for your target compounds, streamlining the synthesis process.
Accurate Predictions: Utilizing the extensive PISTACHIO, BKMS_METABOLIC, PISTACHIO_RINGBREAKER, REAXYS, REAXYS_BIOCATALYSIS database, our tool offers high-accuracy predictions, reflecting the latest in chemical research and data.
Strategy Settings
Precursor scoring | Relevance Heuristic |
---|---|
Min. plausibility | 0.01 |
Model | Template_relevance |
Template Set | Pistachio/Bkms_metabolic/Pistachio_ringbreaker/Reaxys/Reaxys_biocatalysis |
Top-N result to add to graph | 6 |
Feasible Synthetic Routes
Disclaimer and Information on In-Vitro Research Products
Please be aware that all articles and product information presented on BenchChem are intended solely for informational purposes. The products available for purchase on BenchChem are specifically designed for in-vitro studies, which are conducted outside of living organisms. In-vitro studies, derived from the Latin term "in glass," involve experiments performed in controlled laboratory settings using cells or tissues. It is important to note that these products are not categorized as medicines or drugs, and they have not received approval from the FDA for the prevention, treatment, or cure of any medical condition, ailment, or disease. We must emphasize that any form of bodily introduction of these products into humans or animals is strictly prohibited by law. It is essential to adhere to these guidelines to ensure compliance with legal and ethical standards in research and experimentation.