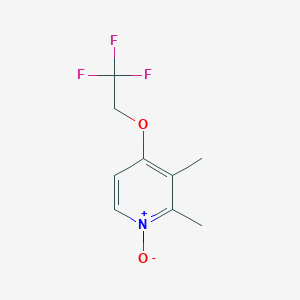
2,3-Dimethyl-4-(2,2,2-trifluoroethoxy)pyridine 1-oxide
Overview
Description
2,3-Dimethyl-4-(2,2,2-trifluoroethoxy)pyridine 1-oxide is an organic compound with the molecular formula C9H10F3NO2. It is a pyridine derivative that features a trifluoroethoxy group and a pyridine N-oxide moiety. This compound is known for its role as an intermediate in the synthesis of various pharmaceuticals, including proton pump inhibitors like lansoprazole .
Mechanism of Action
Target of Action
It is noted that this compound is a useful intermediate in the synthesis of the proton pump inhibitor lansoprazole . Proton pump inhibitors (PPIs) like lansoprazole primarily target the H+/K+ ATPase enzyme system, also known as the gastric proton pump, which is found on the secretory surface of parietal cells and is responsible for the final step of gastric acid production.
Biochemical Analysis
Biochemical Properties
2,3-Dimethyl-4-(2,2,2-trifluoroethoxy)pyridine 1-oxide plays a significant role in biochemical reactions, particularly in the synthesis of pharmaceuticals. This compound interacts with various enzymes and proteins during its synthesis pathway. For instance, it is involved in the production of lansoprazole, where it acts as a precursor . The interactions of this compound with enzymes are crucial for the formation of the final pharmaceutical product.
Cellular Effects
The effects of this compound on cellular processes are primarily observed in its role as an intermediate in drug synthesis. While specific cellular effects of this compound are not extensively documented, its involvement in the synthesis of lansoprazole suggests that it may influence cell signaling pathways and gene expression indirectly through its final product . Lansoprazole, the end product, is known to affect gastric acid secretion by inhibiting the H+/K+ ATPase enzyme in stomach parietal cells.
Molecular Mechanism
At the molecular level, this compound exerts its effects through its interactions with enzymes involved in the synthesis of lansoprazole. The compound’s trifluoroethoxy group is a key functional group that participates in binding interactions with these enzymes, facilitating the conversion of the intermediate to the final product . This process involves enzyme-mediated reactions that lead to the formation of lansoprazole, which then exerts its pharmacological effects.
Temporal Effects in Laboratory Settings
In laboratory settings, the stability and degradation of this compound are important factors to consider. The compound’s stability is crucial for its effective use as an intermediate in drug synthesis. Over time, any degradation of the compound could impact the yield and purity of the final product . Long-term studies on the compound’s stability and its effects on cellular function are essential for optimizing its use in pharmaceutical applications.
Dosage Effects in Animal Models
The effects of this compound at different dosages in animal models are not well-documented. As an intermediate in drug synthesis, its dosage effects are likely studied in the context of the final pharmaceutical product, lansoprazole. High doses of lansoprazole have been associated with toxic effects, and similar studies on the intermediate compound would be necessary to determine any threshold or adverse effects .
Metabolic Pathways
This compound is involved in metabolic pathways related to the synthesis of lansoprazole. The compound interacts with specific enzymes that catalyze its conversion to the final product . These metabolic pathways are essential for the efficient production of lansoprazole, and any disruptions in these pathways could affect the overall synthesis process.
Transport and Distribution
The transport and distribution of this compound within cells and tissues are critical for its role in drug synthesis. The compound must be efficiently transported to the site of enzymatic reactions to facilitate its conversion to lansoprazole . Understanding the transport mechanisms and any binding proteins involved can help optimize the synthesis process.
Subcellular Localization
The subcellular localization of this compound is likely influenced by its interactions with enzymes and other biomolecules involved in drug synthesis. The compound may be directed to specific cellular compartments where these enzymatic reactions occur . Any targeting signals or post-translational modifications that affect its localization can impact its activity and function in the synthesis process.
Preparation Methods
Synthetic Routes and Reaction Conditions: The synthesis of 2,3-Dimethyl-4-(2,2,2-trifluoroethoxy)pyridine 1-oxide typically involves the reaction of 2,3-dimethylpyridine with 2,2,2-trifluoroethanol in the presence of an oxidizing agent. The reaction conditions often include the use of a solvent such as dichloromethane and a catalyst like trifluoroacetic acid. The mixture is stirred at room temperature until the reaction is complete .
Industrial Production Methods: In industrial settings, the production of this compound may involve continuous flow reactors to ensure consistent quality and yield. The process is optimized to minimize waste and reduce production costs. The use of automated systems allows for precise control over reaction parameters, ensuring high purity of the final product .
Types of Reactions:
Oxidation: The compound can undergo further oxidation to form various oxidized derivatives.
Reduction: Reduction reactions can convert the N-oxide group back to the corresponding pyridine.
Substitution: The trifluoroethoxy group can be substituted with other functional groups under appropriate conditions.
Common Reagents and Conditions:
Oxidation: Common oxidizing agents include hydrogen peroxide and m-chloroperbenzoic acid.
Reduction: Reducing agents such as sodium borohydride or lithium aluminum hydride are used.
Substitution: Reagents like alkyl halides or acyl chlorides can be employed for substitution reactions.
Major Products:
Oxidation: Oxidized pyridine derivatives.
Reduction: 2,3-Dimethyl-4-(2,2,2-trifluoroethoxy)pyridine.
Substitution: Various substituted pyridine derivatives depending on the substituent introduced.
Scientific Research Applications
2,3-Dimethyl-4-(2,2,2-trifluoroethoxy)pyridine 1-oxide is widely used in scientific research due to its versatility:
Chemistry: It serves as an intermediate in the synthesis of complex organic molecules and pharmaceuticals.
Biology: The compound is used in studies involving enzyme inhibition and receptor binding.
Medicine: It is a key intermediate in the production of proton pump inhibitors, which are used to treat conditions like gastroesophageal reflux disease.
Industry: The compound is utilized in the development of agrochemicals and other industrial chemicals.
Comparison with Similar Compounds
2,3-Dimethyl-4-(2,2,2-trifluoroethoxy)pyridine: Lacks the N-oxide group.
2,3-Dimethyl-4-(2,2,2-trifluoroethoxy)pyridine-N-oxide: Similar structure but different functional groups.
Lansoprazole: A proton pump inhibitor that uses 2,3-Dimethyl-4-(2,2,2-trifluoroethoxy)pyridine 1-oxide as an intermediate.
Uniqueness: this compound is unique due to its specific combination of functional groups, which confer distinct chemical properties and reactivity. Its role as an intermediate in the synthesis of proton pump inhibitors highlights its importance in medicinal chemistry .
Properties
IUPAC Name |
2,3-dimethyl-1-oxido-4-(2,2,2-trifluoroethoxy)pyridin-1-ium | |
---|---|---|
Source | PubChem | |
URL | https://pubchem.ncbi.nlm.nih.gov | |
Description | Data deposited in or computed by PubChem | |
InChI |
InChI=1S/C9H10F3NO2/c1-6-7(2)13(14)4-3-8(6)15-5-9(10,11)12/h3-4H,5H2,1-2H3 | |
Source | PubChem | |
URL | https://pubchem.ncbi.nlm.nih.gov | |
Description | Data deposited in or computed by PubChem | |
InChI Key |
GMSURXZOJDDQEF-UHFFFAOYSA-N | |
Source | PubChem | |
URL | https://pubchem.ncbi.nlm.nih.gov | |
Description | Data deposited in or computed by PubChem | |
Canonical SMILES |
CC1=C(C=C[N+](=C1C)[O-])OCC(F)(F)F | |
Source | PubChem | |
URL | https://pubchem.ncbi.nlm.nih.gov | |
Description | Data deposited in or computed by PubChem | |
Molecular Formula |
C9H10F3NO2 | |
Source | PubChem | |
URL | https://pubchem.ncbi.nlm.nih.gov | |
Description | Data deposited in or computed by PubChem | |
Molecular Weight |
221.18 g/mol | |
Source | PubChem | |
URL | https://pubchem.ncbi.nlm.nih.gov | |
Description | Data deposited in or computed by PubChem | |
Synthesis routes and methods
Procedure details
Q1: What is significant about the synthesis method described in the research?
A1: The research presents a novel approach to synthesizing 2,3-Dimethyl-4-(2,2,2-trifluoroethoxy)pyridine 1-oxide. The key features of this method are:
Disclaimer and Information on In-Vitro Research Products
Please be aware that all articles and product information presented on BenchChem are intended solely for informational purposes. The products available for purchase on BenchChem are specifically designed for in-vitro studies, which are conducted outside of living organisms. In-vitro studies, derived from the Latin term "in glass," involve experiments performed in controlled laboratory settings using cells or tissues. It is important to note that these products are not categorized as medicines or drugs, and they have not received approval from the FDA for the prevention, treatment, or cure of any medical condition, ailment, or disease. We must emphasize that any form of bodily introduction of these products into humans or animals is strictly prohibited by law. It is essential to adhere to these guidelines to ensure compliance with legal and ethical standards in research and experimentation.