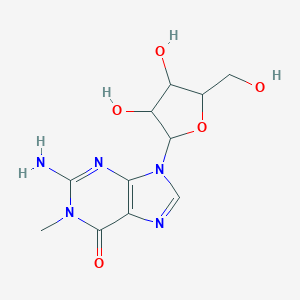
1-Methylguanosine
Overview
Description
N1-Methylguanosine is a methylated nucleoside derived from guanosine. It is characterized by the addition of a methyl group at the nitrogen-1 position of the guanine base. This compound is a significant biomarker in various biological processes and has been studied extensively for its role in RNA modification and potential implications in disease states .
Mechanism of Action
Target of Action
1-Methylguanosine is a methylated nucleoside originating from RNA degradation . The primary targets of this compound are various RNA molecules, including messenger RNA (mRNA), ribosomal RNA (rRNA), microRNA (miRNA), and transfer RNA (tRNA) . The m7G methyltransferases, such as METTL1, WDR4, RNMT, RAM, WBSCR22, and TMRT112, install the m7G modification on these target RNA molecules .
Mode of Action
The mode of action of this compound involves its interaction with its targets, leading to changes in their function. For instance, the METTL1/WDR4 complex increases the abundance of tRNA with m7G modifications, especially tRNA-Arg (anticodon TCT) 4–1 (Arg-TCT-4–1), which affects the translation of mRNA enriched with AGA codons by decreasing ribosome pausing efficacy, particularly in cell cycle progression genes .
Biochemical Pathways
this compound is involved in the modification of RNA molecules, which plays a crucial role in various biochemical pathways. The core structural feature of the mRNA cap is an N7-methylguanosine moiety linked by a 5′–5′ triphosphate chain to the first transcribed nucleotide . This modification actively participates in biological and pathological functions by affecting the metabolism of various RNA molecules .
Pharmacokinetics
It’s known that the compound is a methylated nucleoside originating from rna degradation .
Result of Action
The molecular and cellular effects of this compound’s action are primarily related to the regulation of RNA metabolism. By modifying RNA molecules, this compound can influence the translation of mRNA, thereby affecting gene expression and cellular functions .
Biochemical Analysis
Biochemical Properties
1-Methylguanosine interacts with various enzymes, proteins, and other biomolecules. It is modified by the m1A methyltransferases, or “writers”, such as TRMT6, TRMT61A, TRMT61B, TRMT10C, NML . These interactions play a crucial role in the regulation of various biological functions and activities, especially in processes like cancer cell invasion, proliferation, and cell cycle regulation .
Cellular Effects
This compound has significant effects on various types of cells and cellular processes. It influences cell function by affecting RNA translation and stability . It plays a pivotal role in modulating tumor immunity and mediating various biological behaviors, encompassing proliferation, invasion, metastasis, etc .
Temporal Effects in Laboratory Settings
The effects of this compound change over time in laboratory settings. It is critical for decoding processes and protein translation
Metabolic Pathways
This compound is involved in several metabolic pathways. It is a part of the this compound metabolic process
Subcellular Localization
The subcellular localization of this compound is primarily in the nucleus
Preparation Methods
Synthetic Routes and Reaction Conditions: N1-Methylguanosine can be synthesized through several chemical routes. One common method involves the methylation of guanosine using methyl iodide in the presence of a base such as sodium hydride. The reaction typically occurs in an anhydrous solvent like dimethylformamide at elevated temperatures to ensure complete methylation.
Industrial Production Methods: Industrial production of Nthis compound often involves large-scale methylation reactions using automated reactors. The process is optimized for high yield and purity, employing rigorous purification techniques such as high-performance liquid chromatography to isolate the desired product.
Chemical Reactions Analysis
Types of Reactions: N1-Methylguanosine undergoes various chemical reactions, including:
Oxidation: It can be oxidized to form N1-methylguanine derivatives.
Reduction: Reduction reactions can lead to the formation of dihydro-Nthis compound.
Substitution: Nthis compound can participate in nucleophilic substitution reactions, where the methyl group can be replaced by other functional groups.
Common Reagents and Conditions:
Oxidation: Common oxidizing agents include potassium permanganate and hydrogen peroxide.
Reduction: Reducing agents such as sodium borohydride are typically used.
Substitution: Nucleophiles like thiols and amines are employed under basic conditions.
Major Products: The major products formed from these reactions include various methylated and demethylated guanosine derivatives, which are often studied for their biological activity and potential therapeutic applications .
Scientific Research Applications
N1-Methylguanosine has a wide range of scientific research applications:
Chemistry: It is used as a model compound to study methylation reactions and nucleoside chemistry.
Biology: Nthis compound is a key player in RNA modification, influencing RNA stability, splicing, and translation.
Medicine: Elevated levels of Nthis compound in urine are associated with certain cancers, making it a potential biomarker for cancer diagnosis and prognosis.
Comparison with Similar Compounds
N1-Methylguanosine is unique among methylated nucleosides due to its specific methylation site and biological effects. Similar compounds include:
N7-Methylguanosine: Methylated at the nitrogen-7 position, it plays a role in mRNA capping and stability.
N2,2-Dimethylguanosine: Methylated at the nitrogen-2 position, it is involved in tRNA modification and function.
N1-Methyladenosine: Methylated at the nitrogen-1 position of adenosine, it is another important RNA modification with distinct biological roles.
Nthis compound stands out due to its specific impact on RNA structure and function, making it a valuable compound for research in various scientific fields.
Properties
IUPAC Name |
2-amino-9-[3,4-dihydroxy-5-(hydroxymethyl)oxolan-2-yl]-1-methylpurin-6-one | |
---|---|---|
Details | Computed by Lexichem TK 2.7.0 (PubChem release 2021.05.07) | |
Source | PubChem | |
URL | https://pubchem.ncbi.nlm.nih.gov | |
Description | Data deposited in or computed by PubChem | |
InChI |
InChI=1S/C11H15N5O5/c1-15-9(20)5-8(14-11(15)12)16(3-13-5)10-7(19)6(18)4(2-17)21-10/h3-4,6-7,10,17-19H,2H2,1H3,(H2,12,14) | |
Details | Computed by InChI 1.0.6 (PubChem release 2021.05.07) | |
Source | PubChem | |
URL | https://pubchem.ncbi.nlm.nih.gov | |
Description | Data deposited in or computed by PubChem | |
InChI Key |
UTAIYTHAJQNQDW-UHFFFAOYSA-N | |
Details | Computed by InChI 1.0.6 (PubChem release 2021.05.07) | |
Source | PubChem | |
URL | https://pubchem.ncbi.nlm.nih.gov | |
Description | Data deposited in or computed by PubChem | |
Canonical SMILES |
CN1C(=O)C2=C(N=C1N)N(C=N2)C3C(C(C(O3)CO)O)O | |
Details | Computed by OEChem 2.3.0 (PubChem release 2021.05.07) | |
Source | PubChem | |
URL | https://pubchem.ncbi.nlm.nih.gov | |
Description | Data deposited in or computed by PubChem | |
Molecular Formula |
C11H15N5O5 | |
Details | Computed by PubChem 2.1 (PubChem release 2021.05.07) | |
Source | PubChem | |
URL | https://pubchem.ncbi.nlm.nih.gov | |
Description | Data deposited in or computed by PubChem | |
DSSTOX Substance ID |
DTXSID00862842 | |
Record name | 2-Amino-1-methyl-9-pentofuranosyl-1,9-dihydro-6H-purin-6-one | |
Source | EPA DSSTox | |
URL | https://comptox.epa.gov/dashboard/DTXSID00862842 | |
Description | DSSTox provides a high quality public chemistry resource for supporting improved predictive toxicology. | |
Molecular Weight |
297.27 g/mol | |
Details | Computed by PubChem 2.1 (PubChem release 2021.05.07) | |
Source | PubChem | |
URL | https://pubchem.ncbi.nlm.nih.gov | |
Description | Data deposited in or computed by PubChem | |
CAS No. |
2140-65-0 | |
Record name | 1-Methylguanosine | |
Source | DTP/NCI | |
URL | https://dtp.cancer.gov/dtpstandard/servlet/dwindex?searchtype=NSC&outputformat=html&searchlist=70897 | |
Description | The NCI Development Therapeutics Program (DTP) provides services and resources to the academic and private-sector research communities worldwide to facilitate the discovery and development of new cancer therapeutic agents. | |
Explanation | Unless otherwise indicated, all text within NCI products is free of copyright and may be reused without our permission. Credit the National Cancer Institute as the source. | |
Retrosynthesis Analysis
AI-Powered Synthesis Planning: Our tool employs the Template_relevance Pistachio, Template_relevance Bkms_metabolic, Template_relevance Pistachio_ringbreaker, Template_relevance Reaxys, Template_relevance Reaxys_biocatalysis model, leveraging a vast database of chemical reactions to predict feasible synthetic routes.
One-Step Synthesis Focus: Specifically designed for one-step synthesis, it provides concise and direct routes for your target compounds, streamlining the synthesis process.
Accurate Predictions: Utilizing the extensive PISTACHIO, BKMS_METABOLIC, PISTACHIO_RINGBREAKER, REAXYS, REAXYS_BIOCATALYSIS database, our tool offers high-accuracy predictions, reflecting the latest in chemical research and data.
Strategy Settings
Precursor scoring | Relevance Heuristic |
---|---|
Min. plausibility | 0.01 |
Model | Template_relevance |
Template Set | Pistachio/Bkms_metabolic/Pistachio_ringbreaker/Reaxys/Reaxys_biocatalysis |
Top-N result to add to graph | 6 |
Feasible Synthetic Routes
Disclaimer and Information on In-Vitro Research Products
Please be aware that all articles and product information presented on BenchChem are intended solely for informational purposes. The products available for purchase on BenchChem are specifically designed for in-vitro studies, which are conducted outside of living organisms. In-vitro studies, derived from the Latin term "in glass," involve experiments performed in controlled laboratory settings using cells or tissues. It is important to note that these products are not categorized as medicines or drugs, and they have not received approval from the FDA for the prevention, treatment, or cure of any medical condition, ailment, or disease. We must emphasize that any form of bodily introduction of these products into humans or animals is strictly prohibited by law. It is essential to adhere to these guidelines to ensure compliance with legal and ethical standards in research and experimentation.