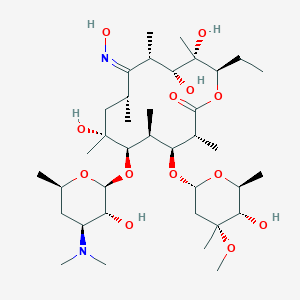
(9Z)-Erythromycin A Oxime
Overview
Description
(9Z)-Erythromycin A Oxime is a geometric isomer of erythromycin A oxime, a key intermediate in synthesizing macrolide antibiotics such as clarithromycin and azithromycin . Historically, the (9Z)-isomer was challenging to isolate due to its thermodynamic instability compared to the (9E)-isomer . However, advancements in isomerization techniques, such as using lithium hydroxide in ethanol, enabled its isolation as a stable crystalline compound .
Physical Properties (from experimental data):
- Molecular formula: C₃₇H₆₈N₂O₁₃
- Melting point: 155–165°C
- Boiling point: 841.0 ± 65.0 °C
- LogP: 2.05 (indicating moderate lipophilicity)
- Solubility: Highly soluble in polar solvents like ethanol and methanol .
The (9Z)-isomer retains antibacterial activity comparable to erythromycin A and serves as a substrate for synthesizing novel derivatives .
Preparation Methods
Synthetic Routes and Reaction Conditions
Erythromycin oxime is typically synthesized by reacting erythromycin A with hydroxylamine in the presence of an organic solvent and an acid catalyst. One common method involves using isopropyl alcohol as the solvent and acetic acid as the catalyst. The reaction is carried out at elevated temperatures, typically around 58°C for 72 hours or 70°C for 24 hours .
Industrial Production Methods
In industrial settings, the preparation of erythromycin oxime involves a two-step process. First, erythromycin thiocyanate is reacted with hydroxylamine hydrochloride in an organic solvent to form erythromycin oxime thiocyanate. This intermediate is then neutralized with an alkali in another organic solvent to yield erythromycin oxime .
Chemical Reactions Analysis
Isomerization of (9E)- to (9Z)-Erythromycin A Oxime
The (9E)-isomer, initially formed during oximation, is isomerized to the (9Z)-isomer via:
- Base-catalyzed isomerization : Lithium hydroxide in alcoholic solvents converts the (9E)-isomer to the (9Z)-isomer .
- Thermodynamic control : The (9Z)-isomer is thermodynamically favored due to reduced steric hindrance between the hydroxyimino group and adjacent substituents .
Table 2: E/Z Isomer Ratios in Synthesis
Catalyst/Solvent | E/Z Ratio | Reference |
---|---|---|
Acetic acid/isopropanol | >6.0 | |
Formic acid/ethanol | >5.5 |
Beckmann Rearrangement
The (9Z)-oxime undergoes Beckmann rearrangement under acidic conditions, forming 8a-aza-8a-homoerythromycin derivatives (e.g., azithromycin precursors) .
- Mechanism : The oxime group (C=N-OH) rearranges to form a lactam ring via cleavage of the C-N bond .
- Key Products :
Table 3: Beckmann Rearrangement Products
Product | Structure Characteristics | Reference |
---|---|---|
8a-aza-8a-homoerythromycin-9,12-iminoether | Lactam ring with azide functionality | |
8a-lactam | Cyclic amide ring |
Oximation and Substitution Reactions
Derivatives of this compound are synthesized via:
Table 4: Antimicrobial Activity of Derivatives
Derivative Type | Activity vs. Erythromycin A | Reference |
---|---|---|
Ether oximes | Comparable | |
Ester oximes | Enhanced in vivo |
Ring-Opening Reactions
Treatment of this compound with hydroxylamine hydrochloride in methanol cleaves the C9/9a-N bond, forming seco-oximes .
- Mechanism : Hydroxylamine attacks the imino bond, leading to bond cleavage and formation of a linear oxime .
- Applications : Formation of novel azalide structures for drug development .
Pharmacokinetic and Stability Studies
Scientific Research Applications
Chemical Structure and Synthesis
The synthesis of (9Z)-Erythromycin A Oxime typically involves the reaction of erythromycin with hydroxylamine under acidic or basic conditions, leading to the introduction of the hydroxyimino group at the C-9 position. This modification plays a significant role in altering the antibiotic properties and pharmacokinetics of the compound.
Scientific Research Applications
This compound has diverse applications across various scientific fields, including chemistry, biology, medicine, and industry. Below are detailed insights into its applications:
Chemistry
- Intermediate in Synthesis : It serves as a critical intermediate in the synthesis of other macrolide antibiotics, such as clarithromycin and azithromycin. These derivatives often exhibit improved efficacy and pharmacokinetic profiles compared to erythromycin.
Biology
- Studying Protein Synthesis : this compound is utilized as a tool for investigating bacterial protein synthesis mechanisms and resistance pathways. Its ability to inhibit protein synthesis by binding to the 50S ribosomal subunit makes it valuable for research on antibiotic resistance.
Medicine
- Antibacterial Activity : The compound retains significant antibacterial activity against various Gram-positive bacteria and some Gram-negative strains. Its structural modifications may enhance its effectiveness against resistant bacterial strains, making it relevant in clinical settings.
- Pharmacological Interactions : As a metabolite of Roxithromycin, this compound has been studied for its pharmacokinetics and potential interactions with cytochrome P450 enzymes, which are crucial for understanding drug-drug interactions that could affect therapeutic outcomes.
Industry
- Pharmaceutical Formulations : It is employed in the production of various pharmaceutical formulations, including nanoparticles and liposomes, which enhance drug delivery and bioavailability. This application is particularly important for improving the therapeutic efficacy of antibiotics in clinical use.
Case Studies
Several studies have documented the applications and effects of this compound:
Case Study 1: Stability Improvement
A study by Brain et al. (1989) demonstrated that converting erythromycin to its oxime derivative significantly improves its stability in acidic environments, such as the stomach. This enhancement potentially leads to better absorption and efficacy when administered orally.
Case Study 2: Antibacterial Efficacy
Research has shown that this compound exhibits effective antibacterial activity against resistant strains of bacteria. In vitro studies indicate that it can inhibit bacterial growth at therapeutic concentrations while demonstrating lower toxicity compared to traditional erythromycin.
Case Study 3: Pharmacokinetic Interactions
Investigations into the pharmacokinetic profile of this compound have revealed that it interacts with various metabolic pathways in humans. Understanding these interactions is essential for optimizing dosage regimens and minimizing adverse effects during treatment.
Comparative Analysis with Other Macrolides
Compound Name | Structural Differences | Unique Features |
---|---|---|
Erythromycin A | No hydroxyimino group | Original compound; foundational macrolide antibiotic |
Roxithromycin | Contains a methoxy group | Improved pharmacokinetics over erythromycin |
Clarithromycin | Methyl substitution at C-6 | Broader spectrum of activity against respiratory pathogens |
Azithromycin | Addition of a nitrogen atom | Extended half-life and better tissue penetration |
This compound | Hydroxyimino group at C-9 | Enhanced antibacterial properties; potential resistance advantages |
Mechanism of Action
Erythromycin oxime exerts its effects by binding to the 50S ribosomal subunit of bacteria, thereby inhibiting protein synthesis. This binding blocks the translocation of peptides, preventing the elongation of the protein chain. As a result, bacterial growth is inhibited, leading to the bacteriostatic effect of erythromycin oxime .
Comparison with Similar Compounds
(9E)-Erythromycin A Oxime
- Structural Difference : The (9E)-isomer has the oxime group in the trans configuration, while the (9Z)-isomer adopts the cis configuration .
- Synthesis: The (9E)-isomer is the primary product of erythromycin A reacting with hydroxylamine, whereas the (9Z)-isomer requires base-catalyzed isomerization (e.g., LiOH in ethanol) .
- Stability : The (9E)-isomer is thermodynamically favored and more stable under standard conditions .
- Biological Activity : Both isomers exhibit similar in vitro antibacterial activity against Gram-positive bacteria (e.g., Staphylococcus aureus), with MIC values ranging 0.25–4 µg/mL .
6-O-Methylerythromycin A 9-Oxime (Clarithromycin Intermediate)
- Structural Modification : The 6-OH group of erythromycin A is methylated, enhancing acid stability and bioavailability .
- Isomer Control : Industrial synthesis prioritizes the (9E)-isomer due to challenges in separating (9Z)-impurities. Chromatographic methods are required for high-purity (9E)-isomer production .
- Activity : 6-O-methyl-(9E)-oxime derivatives show improved pharmacokinetics over erythromycin A, with longer half-lives and higher tissue penetration .
Roxithromycin
- Structural Basis : Derived from (9E)-erythromycin oxime via alkylation of the oxime group with methoxyethoxymethyl ether .
- Advantages : Enhanced acid stability and oral bioavailability compared to erythromycin A. Tissue concentrations are 2–4× higher than plasma levels .
- Limitation : Slightly reduced in vitro potency compared to erythromycin A .
Azithromycin
- Synthesis : Produced via Beckmann rearrangement of erythromycin A oxime, expanding the macrolide ring to 15 members .
- Pharmacokinetics : Superior tissue distribution and half-life (68 hours) compared to erythromycin A (1.5 hours) .
- Activity : Broad-spectrum coverage, including atypical pathogens like Chlamydia .
Key Data Tables
Table 1: Physicochemical Comparison
Property | (9Z)-Erythromycin A Oxime | (9E)-Erythromycin A Oxime | 6-O-Methyl-(9E)-Oxime |
---|---|---|---|
Melting Point (°C) | 155–165 | 160–170 | 220–225 |
LogP | 2.05 | 2.10 | 1.98 |
Solubility (Ethanol) | High | High | Moderate |
Configuration | Z (cis) | E (trans) | E (trans) |
Biological Activity
(9Z)-Erythromycin A oxime is a derivative of erythromycin, a macrolide antibiotic widely used for its antibacterial properties. The biological activity of this compound has been the subject of extensive research, particularly regarding its synthesis, structure-activity relationships, and potential therapeutic applications.
Synthesis and Structural Characteristics
The synthesis of this compound involves the isomerization of the (9E)-isomer to the (9Z)-form, typically using strong bases such as lithium hydroxide in alcoholic solvents . The (9Z)-oxime is characterized by a hydroxyimino group at the 9-position, which influences its biological activity and stability compared to other erythromycin derivatives.
Table 1: Comparison of Erythromycin Derivatives
Compound | Structure Characteristics | Biological Activity |
---|---|---|
Erythromycin A | Lactone ring with 14-membered macrolide | Broad-spectrum antibiotic |
(9E)-Erythromycin A Oxime | Hydroxyimino group at position 9 | Reduced stability in acidic conditions |
This compound | Hydroxyimino group at position 9 | Enhanced stability; potential for better bioavailability |
Biological Activity
The biological activity of this compound has been evaluated in various studies. It exhibits significant antibacterial properties, particularly against Gram-positive bacteria. Research indicates that the oxime modification enhances its stability and may improve oral bioavailability compared to standard erythromycin .
Antibacterial Efficacy
In vitro studies have demonstrated that this compound possesses comparable antibacterial activity to azithromycin, a well-known macrolide antibiotic. The compound shows effectiveness against various strains of bacteria, including resistant strains of Staphylococcus aureus .
Case Studies
- Stability and Bioavailability : A study highlighted that the oximation of erythromycin A improves its stability in gastric conditions, which is crucial for oral administration. The (9Z)-isomer was found to be less reactive in acidic environments than its (9E) counterpart, leading to improved pharmacokinetic profiles .
- Comparative Antimicrobial Activity : Research comparing various ether oxime derivatives of erythromycin revealed that while both stereoisomers exhibit antimicrobial properties, the (9Z) form showed enhanced activity against certain bacterial strains due to its structural attributes .
This compound functions by inhibiting bacterial protein synthesis. It binds to the 50S ribosomal subunit, preventing the translocation process during translation, thereby halting bacterial growth and replication. This mechanism is consistent with other macrolides but is influenced by the specific structural modifications present in the oxime derivative.
Q & A
Basic Research Questions
Q. What are the validated chromatographic methods for analyzing (9Z)-Erythromycin A Oxime and its related substances?
A reverse-phase liquid chromatography (RP-LC) method using an Inertsil C18 ODS column with a mobile phase of phosphate buffer (0.02 M, pH 6.5) and acetonitrile (40:60 v/v) under isocratic elution has been validated for separating this compound from its isomers and degradation products. UV detection at 215 nm ensures specificity for erythromycin A oxime, erythromycin A-6,9-hemiketal, and spiroketal derivatives . Optimization of pH and mobile phase composition is critical to resolving structural analogs, as highlighted in studies comparing HPLC instrument systems .
Q. How do experimental conditions influence the synthesis of this compound?
The oxime derivative is synthesized via oximation of erythromycin A using hydroxylamine under controlled pH and temperature. Key parameters include reaction time (typically 4–6 hours) and purification steps to minimize byproducts like the (9E)-isomer. Studies emphasize the importance of monitoring reaction progress via thin-layer chromatography (TLC) or HPLC to ensure regioselectivity .
Q. What stability challenges are associated with this compound in analytical samples?
this compound is prone to degradation under acidic or alkaline conditions, forming hemiketals and spiroketals. Stability studies recommend storing samples at 4°C in neutral buffers and avoiding prolonged exposure to light. Analytical methods must account for these degradation pathways during method validation .
Advanced Research Questions
Q. How can researchers resolve the (9Z) and (9E) isomers of Erythromycin A Oxime during synthesis?
Isomer separation requires chiral chromatography or recrystallization techniques. A study using a Chiralpak IC column with a hexane-ethanol mobile phase achieved baseline resolution, with the (9Z)-isomer eluting earlier due to its lower polarity. Nuclear magnetic resonance (NMR) and circular dichroism (CD) spectroscopy are critical for confirming stereochemistry .
Q. What computational methods are employed to predict the bioactivity of this compound derivatives?
Density functional theory (DFT) calculations and molecular docking simulations are used to model interactions between the oxime moiety and bacterial ribosomes. These studies highlight the importance of the (9Z) configuration in maintaining hydrogen bonding with 23S rRNA, correlating with macrolide resistance mitigation .
Q. How do structural modifications of this compound impact its pharmacokinetic properties?
Derivatization at the C-6 and C-12 positions (e.g., methylation or glycosylation) alters lipophilicity and metabolic stability. Pharmacokinetic studies in rodent models show that 6-O-methylation increases oral bioavailability by reducing first-pass metabolism, while C-12 modifications enhance tissue penetration .
Q. Methodological Guidance
Q. What statistical approaches are recommended for analyzing bioactivity data in this compound studies?
Dose-response relationships should be modeled using nonlinear regression (e.g., Hill equation) to calculate IC50 values. For comparative studies, ANOVA with post-hoc Tukey tests is recommended to assess significance between derivatives. Ensure sample sizes are justified via power analysis to avoid Type II errors .
Q. How should researchers design experiments to evaluate the environmental stability of this compound?
Use accelerated stability testing under ICH guidelines (e.g., 40°C/75% RH for 6 months) with HPLC-MS monitoring. Include control samples spiked with degradation markers (e.g., erythromycin A enol ether) to validate method robustness. Data should be analyzed using kinetic models (e.g., Arrhenius equation) to predict shelf life .
Q. Data Contradiction and Validation
Q. How to address discrepancies in reported antibacterial activity of this compound across studies?
Variability often arises from differences in bacterial strains, assay conditions (e.g., pH, inoculum size), or isomer purity. Researchers should cross-validate findings using CLSI/MIC protocols and report detailed experimental conditions, including batch-specific HPLC purity data .
Q. What validation parameters are critical for ensuring reproducibility in this compound synthesis?
Key parameters include:
Properties
IUPAC Name |
(3R,4S,5S,6R,7R,9R,10Z,11S,12R,13S,14R)-6-[(2S,3R,4S,6R)-4-(dimethylamino)-3-hydroxy-6-methyloxan-2-yl]oxy-14-ethyl-7,12,13-trihydroxy-10-hydroxyimino-4-[(2R,4R,5S,6S)-5-hydroxy-4-methoxy-4,6-dimethyloxan-2-yl]oxy-3,5,7,9,11,13-hexamethyl-oxacyclotetradecan-2-one | |
---|---|---|
Source | PubChem | |
URL | https://pubchem.ncbi.nlm.nih.gov | |
Description | Data deposited in or computed by PubChem | |
InChI |
InChI=1S/C37H68N2O13/c1-14-25-37(10,45)30(41)20(4)27(38-46)18(2)16-35(8,44)32(52-34-28(40)24(39(11)12)15-19(3)48-34)21(5)29(22(6)33(43)50-25)51-26-17-36(9,47-13)31(42)23(7)49-26/h18-26,28-32,34,40-42,44-46H,14-17H2,1-13H3/b38-27-/t18-,19-,20+,21+,22-,23+,24+,25-,26+,28-,29+,30-,31+,32-,34+,35-,36-,37-/m1/s1 | |
Source | PubChem | |
URL | https://pubchem.ncbi.nlm.nih.gov | |
Description | Data deposited in or computed by PubChem | |
InChI Key |
KYTWXIARANQMCA-PGYIPVOXSA-N | |
Source | PubChem | |
URL | https://pubchem.ncbi.nlm.nih.gov | |
Description | Data deposited in or computed by PubChem | |
Canonical SMILES |
CCC1C(C(C(C(=NO)C(CC(C(C(C(C(C(=O)O1)C)OC2CC(C(C(O2)C)O)(C)OC)C)OC3C(C(CC(O3)C)N(C)C)O)(C)O)C)C)O)(C)O | |
Source | PubChem | |
URL | https://pubchem.ncbi.nlm.nih.gov | |
Description | Data deposited in or computed by PubChem | |
Isomeric SMILES |
CC[C@@H]1[C@@]([C@@H]([C@H](/C(=N\O)/[C@@H](C[C@@]([C@@H]([C@H]([C@@H]([C@H](C(=O)O1)C)O[C@H]2C[C@@]([C@H]([C@@H](O2)C)O)(C)OC)C)O[C@H]3[C@@H]([C@H](C[C@H](O3)C)N(C)C)O)(C)O)C)C)O)(C)O | |
Source | PubChem | |
URL | https://pubchem.ncbi.nlm.nih.gov | |
Description | Data deposited in or computed by PubChem | |
Molecular Formula |
C37H68N2O13 | |
Source | PubChem | |
URL | https://pubchem.ncbi.nlm.nih.gov | |
Description | Data deposited in or computed by PubChem | |
Molecular Weight |
748.9 g/mol | |
Source | PubChem | |
URL | https://pubchem.ncbi.nlm.nih.gov | |
Description | Data deposited in or computed by PubChem | |
CAS No. |
134931-01-4, 13127-18-9 | |
Record name | Erythromycin, 9-oxime, (9Z)- | |
Source | CAS Common Chemistry | |
URL | https://commonchemistry.cas.org/detail?cas_rn=134931-01-4 | |
Description | CAS Common Chemistry is an open community resource for accessing chemical information. Nearly 500,000 chemical substances from CAS REGISTRY cover areas of community interest, including common and frequently regulated chemicals, and those relevant to high school and undergraduate chemistry classes. This chemical information, curated by our expert scientists, is provided in alignment with our mission as a division of the American Chemical Society. | |
Explanation | The data from CAS Common Chemistry is provided under a CC-BY-NC 4.0 license, unless otherwise stated. | |
Record name | Erythromycin, 9-oxime | |
Source | European Chemicals Agency (ECHA) | |
URL | https://echa.europa.eu/substance-information/-/substanceinfo/100.119.162 | |
Description | The European Chemicals Agency (ECHA) is an agency of the European Union which is the driving force among regulatory authorities in implementing the EU's groundbreaking chemicals legislation for the benefit of human health and the environment as well as for innovation and competitiveness. | |
Explanation | Use of the information, documents and data from the ECHA website is subject to the terms and conditions of this Legal Notice, and subject to other binding limitations provided for under applicable law, the information, documents and data made available on the ECHA website may be reproduced, distributed and/or used, totally or in part, for non-commercial purposes provided that ECHA is acknowledged as the source: "Source: European Chemicals Agency, http://echa.europa.eu/". Such acknowledgement must be included in each copy of the material. ECHA permits and encourages organisations and individuals to create links to the ECHA website under the following cumulative conditions: Links can only be made to webpages that provide a link to the Legal Notice page. | |
Record name | (3R,4S,5S,6R,7R,9R,10Z,11S,12R,13S,14R)-6-{[(2S,3R,4S,6R)-4-(dimethylamino)-3-hydroxy-6-methyltetrahydro-2H-pyran-2-yl]oxy}-14-ethyl-7,12,13-trihydroxy-4-{[(2R,4R,5S,6S)-5-hydroxy-4-methoxy-4,6-dimethyltetrahydro-2H-pyran-2-yl]oxy}-3,5,7,9,11,13-hexamethyloxacyclotetradecane-2,10-dione 10-oxime | |
Source | European Chemicals Agency (ECHA) | |
URL | https://echa.europa.eu/substance-information/-/substanceinfo/100.107.526 | |
Description | The European Chemicals Agency (ECHA) is an agency of the European Union which is the driving force among regulatory authorities in implementing the EU's groundbreaking chemicals legislation for the benefit of human health and the environment as well as for innovation and competitiveness. | |
Explanation | Use of the information, documents and data from the ECHA website is subject to the terms and conditions of this Legal Notice, and subject to other binding limitations provided for under applicable law, the information, documents and data made available on the ECHA website may be reproduced, distributed and/or used, totally or in part, for non-commercial purposes provided that ECHA is acknowledged as the source: "Source: European Chemicals Agency, http://echa.europa.eu/". Such acknowledgement must be included in each copy of the material. ECHA permits and encourages organisations and individuals to create links to the ECHA website under the following cumulative conditions: Links can only be made to webpages that provide a link to the Legal Notice page. | |
Retrosynthesis Analysis
AI-Powered Synthesis Planning: Our tool employs the Template_relevance Pistachio, Template_relevance Bkms_metabolic, Template_relevance Pistachio_ringbreaker, Template_relevance Reaxys, Template_relevance Reaxys_biocatalysis model, leveraging a vast database of chemical reactions to predict feasible synthetic routes.
One-Step Synthesis Focus: Specifically designed for one-step synthesis, it provides concise and direct routes for your target compounds, streamlining the synthesis process.
Accurate Predictions: Utilizing the extensive PISTACHIO, BKMS_METABOLIC, PISTACHIO_RINGBREAKER, REAXYS, REAXYS_BIOCATALYSIS database, our tool offers high-accuracy predictions, reflecting the latest in chemical research and data.
Strategy Settings
Precursor scoring | Relevance Heuristic |
---|---|
Min. plausibility | 0.01 |
Model | Template_relevance |
Template Set | Pistachio/Bkms_metabolic/Pistachio_ringbreaker/Reaxys/Reaxys_biocatalysis |
Top-N result to add to graph | 6 |
Feasible Synthetic Routes
Disclaimer and Information on In-Vitro Research Products
Please be aware that all articles and product information presented on BenchChem are intended solely for informational purposes. The products available for purchase on BenchChem are specifically designed for in-vitro studies, which are conducted outside of living organisms. In-vitro studies, derived from the Latin term "in glass," involve experiments performed in controlled laboratory settings using cells or tissues. It is important to note that these products are not categorized as medicines or drugs, and they have not received approval from the FDA for the prevention, treatment, or cure of any medical condition, ailment, or disease. We must emphasize that any form of bodily introduction of these products into humans or animals is strictly prohibited by law. It is essential to adhere to these guidelines to ensure compliance with legal and ethical standards in research and experimentation.