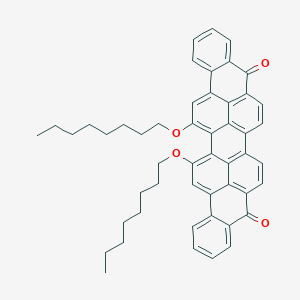
Violanthrone-79
Overview
Description
Violanthrone-79 is a polycyclic aromatic hydrocarbon known for its vibrant color and semiconductive properties. It is an n-channel organic semiconductor used in various electronic applications, including interface control of conventional n-type metal diodes . The compound is characterized by its complex molecular structure, which includes multiple aromatic rings and functional groups.
Mechanism of Action
Target of Action
Violanthrone-79 is primarily an n-channel organic semiconductor . It is used in various applications, including as a model compound for studying the behavior of polar heavy hydrocarbons . The primary targets of this compound are the excitonic states of the molecules it interacts with .
Mode of Action
This compound interacts with its targets through exciton dynamics . In solution, a photo-prepared singlet of this compound exhibits a strong ground-state bleach and stimulated emission feature. When sensitized in its triplet state, it exhibits only a narrow and weak ground-state bleach . This can be explained by the triplet state having absorptions in the same region, with a similar oscillator strength, as the ground state molecule .
Biochemical Pathways
The biochemical pathways affected by this compound involve the interplay of excited singlet excitons and pairs of triplet excitons . This interplay has the potential to transform a number of technologies, including solar photovoltaics, photocatalysis, photodynamic therapy, and biological imaging .
Pharmacokinetics
For instance, in solid films, the excited singlet of this compound is found to survive only 100 ps, giving way to a long-lived transient absorption spectrum with characteristics reminiscent of the triplet in solution . This is interpreted in terms of singlet fission in the solid film .
Result of Action
The result of this compound’s action is the generation of long-lived transient absorption spectra . These spectra are characteristic of the triplet state in solution, suggesting that this compound can induce singlet fission in solid films . This property makes this compound a valuable tool in the study of exciton dynamics and the development of technologies that leverage these dynamics .
Action Environment
The action of this compound is influenced by the environment in which it is placed. For example, the exciton dynamics of this compound differ between solution and solid-state environments . Additionally, the aggregation of this compound and other violanthrone derivatives is thought to occur due to π-π stacking . The nature of this compound aggregates in different solvents is a topic of ongoing research .
Biochemical Analysis
Biochemical Properties
Violanthrone-79 plays a crucial role in biochemical reactions, particularly in the context of its interactions with enzymes, proteins, and other biomolecules. It has been observed to interact with various enzymes involved in metabolic pathways, influencing their activity and stability. For instance, this compound can interact with cytochrome P450 enzymes, affecting their catalytic efficiency and substrate specificity . Additionally, this compound has been shown to bind to certain proteins, altering their conformation and function . These interactions are primarily driven by the compound’s aromatic structure, which facilitates π-π stacking and hydrophobic interactions.
Cellular Effects
This compound exerts significant effects on various types of cells and cellular processes. It has been found to influence cell signaling pathways, gene expression, and cellular metabolism. For example, this compound can modulate the activity of signaling proteins such as kinases and phosphatases, leading to altered phosphorylation states and downstream signaling events . Furthermore, this compound has been reported to affect gene expression by interacting with transcription factors and chromatin-modifying enzymes . These changes in gene expression can result in altered cellular metabolism, impacting processes such as glycolysis and oxidative phosphorylation.
Molecular Mechanism
The molecular mechanism of action of this compound involves several key interactions at the molecular level. This compound can bind to biomolecules through non-covalent interactions such as hydrogen bonding, van der Waals forces, and π-π stacking . These interactions can lead to enzyme inhibition or activation, depending on the specific target. For instance, this compound has been shown to inhibit the activity of certain kinases by binding to their active sites and preventing substrate access . Additionally, this compound can influence gene expression by binding to DNA or interacting with transcription factors, thereby modulating the transcriptional machinery .
Temporal Effects in Laboratory Settings
In laboratory settings, the effects of this compound can change over time due to its stability, degradation, and long-term impact on cellular function. This compound is relatively stable under standard laboratory conditions, but it can undergo degradation when exposed to light or oxidative stress . Long-term studies have shown that this compound can have persistent effects on cellular function, including sustained changes in gene expression and metabolic activity . These temporal effects are important considerations for experimental design and data interpretation.
Dosage Effects in Animal Models
The effects of this compound vary with different dosages in animal models. At low doses, this compound can enhance certain biochemical pathways and improve cellular function . At high doses, this compound can exhibit toxic or adverse effects, including oxidative stress, DNA damage, and apoptosis . These threshold effects highlight the importance of dose optimization in experimental and therapeutic applications.
Metabolic Pathways
This compound is involved in several metabolic pathways, interacting with enzymes and cofactors that regulate metabolic flux and metabolite levels. For example, this compound can be metabolized by cytochrome P450 enzymes, leading to the formation of reactive intermediates that can further interact with cellular components . These metabolic interactions can influence the overall metabolic profile of cells and tissues, affecting processes such as energy production and detoxification.
Transport and Distribution
Within cells and tissues, this compound is transported and distributed through interactions with transporters and binding proteins. This compound can bind to albumin and other plasma proteins, facilitating its distribution in the bloodstream . Additionally, this compound can be taken up by cells through passive diffusion or active transport mechanisms, depending on its concentration and the presence of specific transporters . These transport and distribution dynamics are critical for understanding the bioavailability and pharmacokinetics of this compound.
Subcellular Localization
The subcellular localization of this compound is influenced by its chemical properties and interactions with cellular components. This compound can localize to specific compartments or organelles, such as the nucleus, mitochondria, or endoplasmic reticulum . This localization is often mediated by targeting signals or post-translational modifications that direct this compound to its site of action. The subcellular localization of this compound can impact its activity and function, influencing cellular processes such as gene expression, energy production, and protein synthesis.
Preparation Methods
Synthetic Routes and Reaction Conditions: Violanthrone-79 can be synthesized through a reductive aromatization and functionalization strategy. This involves using low-cost reducing agents such as zinc and sodium dithionite in combination with suitable electrophilic trapping reagents. The process selectively yields violanthrenes functionalized with groups like pivalyloxy, trimethylsiloxy, or methoxy .
Industrial Production Methods: Industrial production of this compound typically involves large-scale synthesis using similar reductive aromatization techniques. The process is optimized for high yield and purity, ensuring the compound’s suitability for electronic applications.
Chemical Reactions Analysis
Types of Reactions: Violanthrone-79 undergoes various chemical reactions, including:
Reduction: The compound can be reduced using agents like zinc and sodium dithionite.
Substitution: Functional groups such as pivalyloxy, trimethylsiloxy, or methoxy can be introduced through electrophilic substitution reactions.
Common Reagents and Conditions:
Reducing Agents: Zinc, sodium dithionite.
Electrophilic Trapping Reagents: Used for introducing functional groups.
Major Products: The major products formed from these reactions are functionalized violanthrenes, which exhibit enhanced solubility and electronic properties .
Scientific Research Applications
Violanthrone-79 has a wide range of scientific research applications:
Chemistry: Used as a starting material for synthesizing substituted violanthrenes.
Biology: Investigated for its potential in biological imaging due to its fluorescence properties.
Medicine: Explored for photodynamic therapy applications.
Industry: Utilized in the production of organic semiconductors for electronic devices.
Comparison with Similar Compounds
- Violanthrone-78
- Isoviolanthrone
- Vat Violet 1 (C.I. 60010)
- Vat Blue 18 (C.I. 59815)
Violanthrone-79 stands out for its unique combination of properties, making it a valuable compound in various scientific and industrial fields.
Biological Activity
Violanthrone-79, a synthetic organic compound, has garnered attention for its diverse biological activities and potential applications in various fields, including medicine, environmental science, and materials science. This article delves into the biological activity of this compound, examining its mechanisms of action, cellular effects, and potential therapeutic uses.
Target Interactions
this compound primarily acts as an n-channel organic semiconductor , influencing excitonic states in the molecules it interacts with. The compound engages in exciton dynamics , which are critical for its biological effects. It has been shown to interact with various biomolecules, including enzymes and proteins, through non-covalent interactions such as hydrogen bonding and π-π stacking.
Biochemical Pathways
The compound affects several biochemical pathways by modulating enzyme activity. Notably, it interacts with cytochrome P450 enzymes , which play a crucial role in drug metabolism and the synthesis of cholesterol, steroids, and other lipids. This interaction can alter the catalytic efficiency and substrate specificity of these enzymes, potentially impacting metabolic processes.
Cellular Effects
This compound exhibits significant effects on cellular functions:
- Gene Expression : Long-term exposure to this compound can lead to sustained changes in gene expression profiles.
- Cell Signaling : It modulates signaling pathways by influencing proteins such as kinases and phosphatases, resulting in altered phosphorylation states that affect downstream signaling events.
- Metabolic Regulation : The compound is involved in regulating metabolic flux by interacting with various cofactors and enzymes, thus influencing metabolite levels within cells.
Pharmacokinetics
This compound demonstrates unique pharmacokinetic properties. In solid films, the excited singlet state of this compound lasts approximately 100 picoseconds before transitioning to a long-lived transient absorption spectrum reminiscent of triplet states observed in solution. This characteristic is crucial for its applications in photodynamic therapy and biological imaging .
Photodynamic Therapy Applications
Research has explored the use of this compound in photodynamic therapy (PDT), where it acts as a photosensitizer. In a study involving cancer cell lines, the compound showed enhanced cytotoxicity when activated by light, leading to increased apoptosis rates compared to untreated controls. This suggests its potential as an effective agent in cancer treatment protocols .
Environmental Impact
Another study investigated the environmental implications of this compound's degradation products. The compound was found to undergo photodegradation under UV light, resulting in less toxic byproducts that could mitigate environmental risks associated with organic pollutants. This property highlights its dual role as both a chemical agent and a potential environmental remediation tool .
Comparative Analysis
To better understand the unique properties of this compound, a comparison with similar compounds is beneficial:
Compound | Biological Activity | Key Features |
---|---|---|
Violanthrone-78 | Similar excitonic properties | Less stable than this compound |
Isoviolanthrone | Limited biological studies available | Structural isomer with different properties |
Vat Violet 1 | Known for dyeing applications | Less emphasis on biological activity |
Vat Blue 18 | Similar to Vat Violet 1 | Primarily used in textiles |
This compound stands out due to its combination of photophysical properties and biochemical interactions that allow for significant biological activity.
Properties
IUPAC Name |
30,34-dioctoxynonacyclo[18.10.2.22,5.03,16.04,13.06,11.017,31.022,27.028,32]tetratriaconta-1(30),2(34),3(16),4(13),5(33),6,8,10,14,17(31),18,20(32),22,24,26,28-hexadecaene-12,21-dione | |
---|---|---|
Source | PubChem | |
URL | https://pubchem.ncbi.nlm.nih.gov | |
Description | Data deposited in or computed by PubChem | |
InChI |
InChI=1S/C50H48O4/c1-3-5-7-9-11-17-27-53-41-29-39-31-19-13-15-21-35(31)49(51)37-25-23-33-34-24-26-38-44-40(32-20-14-16-22-36(32)50(38)52)30-42(54-28-18-12-10-8-6-4-2)48(46(34)44)47(41)45(33)43(37)39/h13-16,19-26,29-30H,3-12,17-18,27-28H2,1-2H3 | |
Source | PubChem | |
URL | https://pubchem.ncbi.nlm.nih.gov | |
Description | Data deposited in or computed by PubChem | |
InChI Key |
LLPQZABTDLOYAL-UHFFFAOYSA-N | |
Source | PubChem | |
URL | https://pubchem.ncbi.nlm.nih.gov | |
Description | Data deposited in or computed by PubChem | |
Canonical SMILES |
CCCCCCCCOC1=C2C3=C(C=CC4=C3C(=C1)C5=CC=CC=C5C4=O)C6=C7C2=C(C=C8C7=C(C=C6)C(=O)C9=CC=CC=C98)OCCCCCCCC | |
Source | PubChem | |
URL | https://pubchem.ncbi.nlm.nih.gov | |
Description | Data deposited in or computed by PubChem | |
Molecular Formula |
C50H48O4 | |
Source | PubChem | |
URL | https://pubchem.ncbi.nlm.nih.gov | |
Description | Data deposited in or computed by PubChem | |
DSSTOX Substance ID |
DTXSID50584562 | |
Record name | 16,17-Bis(octyloxy)anthra[9,1,2-cde]benzo[rst]pentaphene-5,10-dione | |
Source | EPA DSSTox | |
URL | https://comptox.epa.gov/dashboard/DTXSID50584562 | |
Description | DSSTox provides a high quality public chemistry resource for supporting improved predictive toxicology. | |
Molecular Weight |
712.9 g/mol | |
Source | PubChem | |
URL | https://pubchem.ncbi.nlm.nih.gov | |
Description | Data deposited in or computed by PubChem | |
CAS No. |
85652-50-2 | |
Record name | 16,17-Bis(octyloxy)anthra[9,1,2-cde]benzo[rst]pentaphene-5,10-dione | |
Source | EPA DSSTox | |
URL | https://comptox.epa.gov/dashboard/DTXSID50584562 | |
Description | DSSTox provides a high quality public chemistry resource for supporting improved predictive toxicology. | |
Retrosynthesis Analysis
AI-Powered Synthesis Planning: Our tool employs the Template_relevance Pistachio, Template_relevance Bkms_metabolic, Template_relevance Pistachio_ringbreaker, Template_relevance Reaxys, Template_relevance Reaxys_biocatalysis model, leveraging a vast database of chemical reactions to predict feasible synthetic routes.
One-Step Synthesis Focus: Specifically designed for one-step synthesis, it provides concise and direct routes for your target compounds, streamlining the synthesis process.
Accurate Predictions: Utilizing the extensive PISTACHIO, BKMS_METABOLIC, PISTACHIO_RINGBREAKER, REAXYS, REAXYS_BIOCATALYSIS database, our tool offers high-accuracy predictions, reflecting the latest in chemical research and data.
Strategy Settings
Precursor scoring | Relevance Heuristic |
---|---|
Min. plausibility | 0.01 |
Model | Template_relevance |
Template Set | Pistachio/Bkms_metabolic/Pistachio_ringbreaker/Reaxys/Reaxys_biocatalysis |
Top-N result to add to graph | 6 |
Feasible Synthetic Routes
Disclaimer and Information on In-Vitro Research Products
Please be aware that all articles and product information presented on BenchChem are intended solely for informational purposes. The products available for purchase on BenchChem are specifically designed for in-vitro studies, which are conducted outside of living organisms. In-vitro studies, derived from the Latin term "in glass," involve experiments performed in controlled laboratory settings using cells or tissues. It is important to note that these products are not categorized as medicines or drugs, and they have not received approval from the FDA for the prevention, treatment, or cure of any medical condition, ailment, or disease. We must emphasize that any form of bodily introduction of these products into humans or animals is strictly prohibited by law. It is essential to adhere to these guidelines to ensure compliance with legal and ethical standards in research and experimentation.