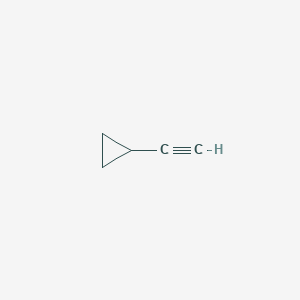
Cyclopropylacetylene
Overview
Description
Cyclopropylacetylene, also known as ethynylcyclopropane, is a hydrocarbon with the chemical formula C₅H₆. Under normal conditions, it is a colorless, flammable liquid. This compound is notable for its use as a precursor in the synthesis of pharmaceuticals and other organic compounds .
Mechanism of Action
Target of Action
Cyclopropylacetylene, also known as Ethynylcyclopropane, is a hydrocarbon with the chemical formula C5H6 . It has been identified as a crucial synthetic intermediate for the antiretroviral and psychotropic drug Efavirenz . Efavirenz is a potent inhibitor of the human immunodeficiency virus type-1 (HIV-1) non-nucleoside reverse transcriptase . Therefore, the primary target of this compound, when used in this context, is the HIV-1 non-nucleoside reverse transcriptase.
Mode of Action
Efavirenz binds to HIV-1 reverse transcriptase, inhibiting the enzyme and preventing the transcription of viral RNA into DNA, a necessary step for the replication of the virus .
Biochemical Pathways
It is a precursor in the synthesis of efavirenz . Efavirenz, in turn, affects the HIV-1 replication pathway by inhibiting the reverse transcriptase enzyme . This inhibition prevents the conversion of viral RNA into DNA, thereby disrupting the viral replication process.
Pharmacokinetics
As a small hydrocarbon, its bioavailability would be influenced by factors such as its lipophilicity, molecular size, and chemical stability .
Result of Action
Efavirenz inhibits the HIV-1 reverse transcriptase enzyme, preventing the transcription of viral RNA into DNA and thereby disrupting the replication of the virus .
Action Environment
As a volatile organic compound, this compound’s stability could be affected by exposure to heat or light .
Preparation Methods
Synthetic Routes and Reaction Conditions: Several methods have been developed for the synthesis of cyclopropylacetylene. One of the earliest methods involves the chlorination of cyclopropylmethylketone with phosphorus pentachloride, followed by double dehydrochlorination in the presence of a strong base such as potassium tert-butoxide in dimethyl sulfoxide . this method yields only about 20-25%.
A more efficient one-pot synthesis involves the reaction of 5-chloro-1-pentyne with n-butyl lithium or n-hexyl lithium in cyclohexane. This reaction proceeds through metalation followed by cyclization, and the product is then isolated by adding an aqueous solution of ammonium chloride .
Industrial Production Methods: An industrially viable method involves dissolving cyclopropylmethyl ketone, a chlorination reagent, an organic base, and a catalyst in an organic solvent. The mixture is stirred at temperatures ranging from 0 to 150°C until the reaction is complete. The product, chlorocyclopropylethylene, is then subjected to further reaction with an alkali to yield this compound .
Chemical Reactions Analysis
Types of Reactions: Cyclopropylacetylene undergoes various types of chemical reactions, including:
Oxidation: It can be oxidized to form cyclopropylacetaldehyde.
Reduction: Reduction reactions can yield cyclopropyl ethane.
Substitution: It can participate in substitution reactions, such as the azide-alkyne Huisgen cycloaddition.
Common Reagents and Conditions:
Oxidation: Common oxidizing agents include potassium permanganate and chromium trioxide.
Reduction: Hydrogen gas in the presence of a palladium catalyst is often used.
Substitution: Copper(I) catalysts are commonly used in cycloaddition reactions.
Major Products:
Oxidation: Cyclopropylacetaldehyde.
Reduction: Cyclopropyl ethane.
Substitution: Various substituted cyclopropyl derivatives, depending on the specific reagents used.
Scientific Research Applications
Cyclopropylacetylene is used extensively in scientific research due to its versatility:
Biology: It is used in the study of enzyme mechanisms and as a probe in biochemical assays.
Industry: It is used in the production of specialty chemicals and materials.
Comparison with Similar Compounds
Cyclopropylacetylene can be compared with other similar compounds such as:
Cyclopropylmethylketone: Used as a precursor in the synthesis of this compound.
Cyclopropylethynylcarbinols: Synthesized from this compound and used in various organic reactions.
Cyclopropyl ethane: A reduced form of this compound.
The uniqueness of this compound lies in its combination of a cyclopropyl ring and an acetylene group, which imparts distinct reactivity and versatility in synthetic applications .
Properties
IUPAC Name |
ethynylcyclopropane | |
---|---|---|
Source | PubChem | |
URL | https://pubchem.ncbi.nlm.nih.gov | |
Description | Data deposited in or computed by PubChem | |
InChI |
InChI=1S/C5H6/c1-2-5-3-4-5/h1,5H,3-4H2 | |
Source | PubChem | |
URL | https://pubchem.ncbi.nlm.nih.gov | |
Description | Data deposited in or computed by PubChem | |
InChI Key |
NPTDXPDGUHAFKC-UHFFFAOYSA-N | |
Source | PubChem | |
URL | https://pubchem.ncbi.nlm.nih.gov | |
Description | Data deposited in or computed by PubChem | |
Canonical SMILES |
C#CC1CC1 | |
Source | PubChem | |
URL | https://pubchem.ncbi.nlm.nih.gov | |
Description | Data deposited in or computed by PubChem | |
Molecular Formula |
C5H6 | |
Source | PubChem | |
URL | https://pubchem.ncbi.nlm.nih.gov | |
Description | Data deposited in or computed by PubChem | |
DSSTOX Substance ID |
DTXSID80217795 | |
Record name | Cyclopropylacetylene | |
Source | EPA DSSTox | |
URL | https://comptox.epa.gov/dashboard/DTXSID80217795 | |
Description | DSSTox provides a high quality public chemistry resource for supporting improved predictive toxicology. | |
Molecular Weight |
66.10 g/mol | |
Source | PubChem | |
URL | https://pubchem.ncbi.nlm.nih.gov | |
Description | Data deposited in or computed by PubChem | |
CAS No. |
6746-94-7 | |
Record name | Cyclopropylacetylene | |
Source | CAS Common Chemistry | |
URL | https://commonchemistry.cas.org/detail?cas_rn=6746-94-7 | |
Description | CAS Common Chemistry is an open community resource for accessing chemical information. Nearly 500,000 chemical substances from CAS REGISTRY cover areas of community interest, including common and frequently regulated chemicals, and those relevant to high school and undergraduate chemistry classes. This chemical information, curated by our expert scientists, is provided in alignment with our mission as a division of the American Chemical Society. | |
Explanation | The data from CAS Common Chemistry is provided under a CC-BY-NC 4.0 license, unless otherwise stated. | |
Record name | Cyclopropylacetylene | |
Source | ChemIDplus | |
URL | https://pubchem.ncbi.nlm.nih.gov/substance/?source=chemidplus&sourceid=0006746947 | |
Description | ChemIDplus is a free, web search system that provides access to the structure and nomenclature authority files used for the identification of chemical substances cited in National Library of Medicine (NLM) databases, including the TOXNET system. | |
Record name | Cyclopropylacetylene | |
Source | EPA DSSTox | |
URL | https://comptox.epa.gov/dashboard/DTXSID80217795 | |
Description | DSSTox provides a high quality public chemistry resource for supporting improved predictive toxicology. | |
Record name | ethynyl cyclopropane | |
Source | European Chemicals Agency (ECHA) | |
URL | https://echa.europa.eu/substance-information/-/substanceinfo/100.102.389 | |
Description | The European Chemicals Agency (ECHA) is an agency of the European Union which is the driving force among regulatory authorities in implementing the EU's groundbreaking chemicals legislation for the benefit of human health and the environment as well as for innovation and competitiveness. | |
Explanation | Use of the information, documents and data from the ECHA website is subject to the terms and conditions of this Legal Notice, and subject to other binding limitations provided for under applicable law, the information, documents and data made available on the ECHA website may be reproduced, distributed and/or used, totally or in part, for non-commercial purposes provided that ECHA is acknowledged as the source: "Source: European Chemicals Agency, http://echa.europa.eu/". Such acknowledgement must be included in each copy of the material. ECHA permits and encourages organisations and individuals to create links to the ECHA website under the following cumulative conditions: Links can only be made to webpages that provide a link to the Legal Notice page. | |
Record name | Cyclopropane, ethynyl | |
Source | European Chemicals Agency (ECHA) | |
URL | https://echa.europa.eu/substance-information/-/substanceinfo/100.120.716 | |
Description | The European Chemicals Agency (ECHA) is an agency of the European Union which is the driving force among regulatory authorities in implementing the EU's groundbreaking chemicals legislation for the benefit of human health and the environment as well as for innovation and competitiveness. | |
Explanation | Use of the information, documents and data from the ECHA website is subject to the terms and conditions of this Legal Notice, and subject to other binding limitations provided for under applicable law, the information, documents and data made available on the ECHA website may be reproduced, distributed and/or used, totally or in part, for non-commercial purposes provided that ECHA is acknowledged as the source: "Source: European Chemicals Agency, http://echa.europa.eu/". Such acknowledgement must be included in each copy of the material. ECHA permits and encourages organisations and individuals to create links to the ECHA website under the following cumulative conditions: Links can only be made to webpages that provide a link to the Legal Notice page. | |
Q1: What is the molecular formula and weight of cyclopropylacetylene?
A1: this compound has a molecular formula of C5H6 and a molecular weight of 66.10 g/mol.
Q2: What spectroscopic data is available for characterizing this compound?
A: Researchers have utilized microwave spectroscopy, electron diffraction, and vibrational spectroscopy to determine the structure of this compound. [, , , ] These techniques have revealed key structural features such as bond lengths and angles.
Q3: Is this compound stable under ambient conditions?
A3: While specific data on long-term stability is limited in the provided research, this compound is typically handled as a reactive compound.
Q4: Can this compound participate in metal-catalyzed reactions?
A: Yes, this compound readily undergoes transformations in the presence of various transition metal catalysts. For instance, gold and gallium catalysts facilitate the annulation reaction of this compound with 1,3-dicarbonyl compounds to yield substituted cyclopentenes. [, ]
Q5: Are there any metal-free reactions involving this compound?
A: Research highlights the use of (C6F5)2BX compounds for metal-free acetylene coupling reactions, including this compound oligomerization. [] This approach offers an alternative pathway for this compound functionalization.
Q6: Have computational methods been employed to study this compound reactions?
A: Density functional theory (DFT) calculations have been instrumental in elucidating the mechanism of nitrosoarene-alkyne cycloadditions, including those involving this compound. [] This approach helps predict reaction pathways and regioselectivity.
Q7: How do structural modifications to this compound impact its reactivity?
A: Substitutions on the cyclopropyl ring or the acetylenic moiety can significantly influence reactivity. For example, 1-ethynylcyclopropylamine and 1-ethynylcyclobutylamine exhibit different reactivity profiles compared to this compound. []
Q8: What analytical techniques are commonly used to characterize this compound and its derivatives?
A: Researchers frequently employ techniques like nuclear magnetic resonance (NMR) spectroscopy, gas chromatography-mass spectrometry (GC-MS), and high-resolution electrospray ionization mass spectrometry (HRESI-MS) to analyze this compound and its reaction products. [, , ]
Disclaimer and Information on In-Vitro Research Products
Please be aware that all articles and product information presented on BenchChem are intended solely for informational purposes. The products available for purchase on BenchChem are specifically designed for in-vitro studies, which are conducted outside of living organisms. In-vitro studies, derived from the Latin term "in glass," involve experiments performed in controlled laboratory settings using cells or tissues. It is important to note that these products are not categorized as medicines or drugs, and they have not received approval from the FDA for the prevention, treatment, or cure of any medical condition, ailment, or disease. We must emphasize that any form of bodily introduction of these products into humans or animals is strictly prohibited by law. It is essential to adhere to these guidelines to ensure compliance with legal and ethical standards in research and experimentation.