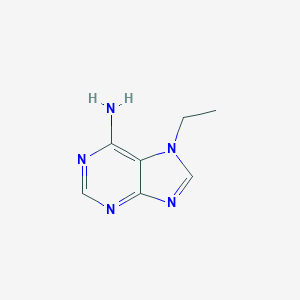
7-Ethyl-7h-purin-6-amine
- Click on QUICK INQUIRY to receive a quote from our team of experts.
- With the quality product at a COMPETITIVE price, you can focus more on your research.
Overview
Description
7-Ethyl-7h-purin-6-amine is a purine derivative with the molecular formula C7H9N5. This compound is structurally related to adenine, one of the four nucleobases in the nucleic acids of DNA and RNA. The ethyl group at the 7th position of the purine ring distinguishes it from other purine derivatives.
Preparation Methods
Synthetic Routes and Reaction Conditions
The synthesis of 7-Ethyl-7h-purin-6-amine typically involves the alkylation of adenine. One common method includes the addition of ethyl iodide to a suspension of adenine and cesium carbonate in dry N,N-dimethylformamide under an inert nitrogen atmosphere. The reaction mixture is heated at 60°C for 7 hours, resulting in the formation of this compound .
Industrial Production Methods
While specific industrial production methods for this compound are not extensively documented, the general approach involves large-scale alkylation reactions similar to the laboratory synthesis. The use of high-purity reagents and controlled reaction conditions ensures the consistent production of the compound.
Chemical Reactions Analysis
Types of Reactions
7-Ethyl-7h-purin-6-amine undergoes various chemical reactions, including:
Substitution Reactions: The ethyl group can be substituted with other alkyl or functional groups under appropriate conditions.
Oxidation and Reduction: The compound can participate in redox reactions, altering its oxidation state and forming different derivatives.
Common Reagents and Conditions
Substitution Reactions: Reagents such as alkyl halides or acyl chlorides can be used in the presence of a base like cesium carbonate.
Oxidation and Reduction: Oxidizing agents like hydrogen peroxide or reducing agents like sodium borohydride can be employed.
Major Products Formed
The major products formed from these reactions depend on the specific reagents and conditions used. For example, substitution reactions can yield various alkylated purine derivatives, while oxidation and reduction reactions can produce different oxidized or reduced forms of the compound.
Scientific Research Applications
7-Ethyl-7h-purin-6-amine has several scientific research applications:
Chemistry: It is used as a building block in the synthesis of more complex purine derivatives.
Biology: The compound is studied for its potential role in nucleic acid interactions and enzyme inhibition.
Medicine: Research explores its potential as an anticancer agent due to its structural similarity to adenine, which can interfere with DNA replication and transcription.
Industry: It is used in the development of pharmaceuticals and agrochemicals.
Mechanism of Action
The mechanism of action of 7-Ethyl-7h-purin-6-amine involves its interaction with nucleic acids and enzymes. By mimicking adenine, it can incorporate into DNA or RNA, potentially disrupting normal cellular processes. This disruption can lead to the inhibition of cell proliferation, making it a candidate for anticancer research .
Comparison with Similar Compounds
Similar Compounds
7-Methyl-7h-purin-6-amine: Similar in structure but with a methyl group instead of an ethyl group.
6-Amino-9-ethyl-9H-purine: Another ethylated purine derivative with the ethyl group at the 9th position.
Uniqueness
7-Ethyl-7h-purin-6-amine is unique due to its specific ethyl substitution at the 7th position, which can influence its chemical reactivity and biological activity compared to other purine derivatives .
Properties
IUPAC Name |
7-ethylpurin-6-amine |
Source
|
---|---|---|
Source | PubChem | |
URL | https://pubchem.ncbi.nlm.nih.gov | |
Description | Data deposited in or computed by PubChem | |
InChI |
InChI=1S/C7H9N5/c1-2-12-4-11-7-5(12)6(8)9-3-10-7/h3-4H,2H2,1H3,(H2,8,9,10) |
Source
|
Source | PubChem | |
URL | https://pubchem.ncbi.nlm.nih.gov | |
Description | Data deposited in or computed by PubChem | |
InChI Key |
HCTWNRRHYBYREK-UHFFFAOYSA-N |
Source
|
Source | PubChem | |
URL | https://pubchem.ncbi.nlm.nih.gov | |
Description | Data deposited in or computed by PubChem | |
Canonical SMILES |
CCN1C=NC2=NC=NC(=C21)N |
Source
|
Source | PubChem | |
URL | https://pubchem.ncbi.nlm.nih.gov | |
Description | Data deposited in or computed by PubChem | |
Molecular Formula |
C7H9N5 |
Source
|
Source | PubChem | |
URL | https://pubchem.ncbi.nlm.nih.gov | |
Description | Data deposited in or computed by PubChem | |
DSSTOX Substance ID |
DTXSID00947143 |
Source
|
Record name | 7-Ethyl-7H-purin-6-amine | |
Source | EPA DSSTox | |
URL | https://comptox.epa.gov/dashboard/DTXSID00947143 | |
Description | DSSTox provides a high quality public chemistry resource for supporting improved predictive toxicology. | |
Molecular Weight |
163.18 g/mol |
Source
|
Source | PubChem | |
URL | https://pubchem.ncbi.nlm.nih.gov | |
Description | Data deposited in or computed by PubChem | |
CAS No. |
24309-36-2 |
Source
|
Record name | 7H-Purin-6-amine, 7-ethyl- | |
Source | ChemIDplus | |
URL | https://pubchem.ncbi.nlm.nih.gov/substance/?source=chemidplus&sourceid=0024309362 | |
Description | ChemIDplus is a free, web search system that provides access to the structure and nomenclature authority files used for the identification of chemical substances cited in National Library of Medicine (NLM) databases, including the TOXNET system. | |
Record name | 7-Ethyl-7H-purin-6-amine | |
Source | EPA DSSTox | |
URL | https://comptox.epa.gov/dashboard/DTXSID00947143 | |
Description | DSSTox provides a high quality public chemistry resource for supporting improved predictive toxicology. | |
Disclaimer and Information on In-Vitro Research Products
Please be aware that all articles and product information presented on BenchChem are intended solely for informational purposes. The products available for purchase on BenchChem are specifically designed for in-vitro studies, which are conducted outside of living organisms. In-vitro studies, derived from the Latin term "in glass," involve experiments performed in controlled laboratory settings using cells or tissues. It is important to note that these products are not categorized as medicines or drugs, and they have not received approval from the FDA for the prevention, treatment, or cure of any medical condition, ailment, or disease. We must emphasize that any form of bodily introduction of these products into humans or animals is strictly prohibited by law. It is essential to adhere to these guidelines to ensure compliance with legal and ethical standards in research and experimentation.