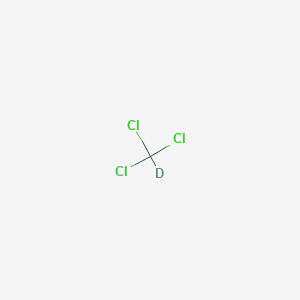
Chloroform-d
Overview
Description
Chloroform-d, also known as deuterated chloroform, is an organic compound with the formula CDCl₃. It is an isotopologue of chloroform (CHCl₃) where the hydrogen atom is replaced by deuterium, a heavier isotope of hydrogen. This compound is widely used as a solvent in nuclear magnetic resonance (NMR) spectroscopy due to its ability to dissolve a wide range of compounds and its minimal interference with the NMR signals .
Mechanism of Action
Target of Action
Chloroform-D, also known as Deuterochloroform, is primarily used as a solvent in Nuclear Magnetic Resonance (NMR) spectroscopy . It is widely employed in high-resolution NMR studies due to its high chemical and isotopic purity . The primary target of this compound is therefore the molecules being studied in NMR analysis.
Mode of Action
This compound interacts with its targets (the molecules being studied) by providing a medium that minimizes interference from water peaks during NMR analysis . This allows for a clearer and more accurate study of the molecules .
Biochemical Pathways
It plays a crucial role in facilitating the study of these pathways by providing a suitable environment for nmr analysis .
Pharmacokinetics
It’s worth noting that this compound has a boiling point of 609 °C and a density of 1500 g/mL at 25 °C . These properties can impact its behavior as a solvent and its suitability for various experimental conditions.
Result of Action
The primary result of this compound’s action as an NMR solvent is the facilitation of clear and accurate NMR analysis. By minimizing interference from water peaks, this compound allows for the detailed study of molecular structures and interactions .
Action Environment
The efficacy and stability of this compound as an NMR solvent can be influenced by various environmental factors. For instance, temperature can affect the density of this compound and thus its performance as a solvent . Additionally, this compound is sensitive to light and can decompose to form phosgene and hydrogen chloride . Therefore, it is typically stored in brown-tinted bottles to slow this process and reduce its acidity .
Biochemical Analysis
Biochemical Properties
Chloroform-D interacts with various biomolecules such as enzymes and proteins. For instance, it has been found to affect the electrical activity of proteinoids, which are thermal proteins that produce hollow microspheres in aqueous solutions .
Cellular Effects
This compound has significant effects on various types of cells and cellular processes. For example, it has been shown to accumulate preferentially in highly disordered lipid domains of cell membranes, suggesting that it could influence cell function by altering membrane properties . Furthermore, this compound exposure can lead to changes in gene expression and cellular metabolism .
Molecular Mechanism
The molecular mechanism of this compound involves its metabolism by two different pathways: oxidative dechlorination leading to the formation of phosgene and a reductive pathway leading to the formation of a reactive free radical . These metabolites can react with cellular components such as fatty acids and phospholipids, leading to lipid peroxidation, which accounts for the hepatotoxic and nephrotoxic effects of this compound .
Temporal Effects in Laboratory Settings
In laboratory settings, the effects of this compound can change over time. For instance, it has been found that this compound can enhance lipid extraction efficiency in microalgae, with the efficiency increasing with the number of freeze-thaw cycles . This suggests that this compound may have long-term effects on cellular function in in vitro or in vivo studies.
Dosage Effects in Animal Models
The effects of this compound can vary with different dosages in animal models. For instance, a study investigating the toxicological and anti-malarial effects of the chloroform fractions of certain medicinal plants in murine models found that all three fractions did not influence mortality, clinical appearance, body weight gain, or necropsy at various doses .
Metabolic Pathways
This compound is involved in various metabolic pathways. During its metabolism, reactive intermediates such as phosgene and dichloromethyl radicals are formed, which can react with cellular components . This can lead to changes in metabolic flux or metabolite levels.
Transport and Distribution
This compound is transported and distributed within cells and tissues. It has been shown to accumulate preferentially in highly disordered lipid domains of cell membranes . This could influence its localization or accumulation within cells.
Subcellular Localization
The subcellular localization of this compound is primarily within the lipid domains of cell membranes . This localization could influence its activity or function within the cell. For instance, its presence in the lipid domains could influence membrane properties, potentially affecting the function of membrane-bound proteins and enzymes .
Preparation Methods
Synthetic Routes and Reaction Conditions: Chloroform-d is typically synthesized by the reaction of hexachloroacetone with deuterium oxide (D₂O) in the presence of pyridine as a catalyst. The reaction proceeds as follows:
O=C(CCl₃)₂ + D₂O → 2 CDCl₃ + CO₂
The large difference in boiling points between the starting material and the product facilitates purification by distillation .
Industrial Production Methods: On an industrial scale, this compound is produced similarly to its laboratory synthesis. The reaction involves hexachloroacetone and deuterium oxide, with pyridine acting as a catalyst. The product is then purified through distillation to obtain high-purity this compound .
Chemical Reactions Analysis
Types of Reactions: Chloroform-d undergoes several types of chemical reactions, including:
Oxidation: In the presence of light and oxygen, this compound can be oxidized to form phosgene (COCl₂), chlorine (Cl₂), and hydrogen chloride (HCl).
Common Reagents and Conditions:
Oxidation: Light and oxygen.
Hydrolysis: Aqueous potassium hydroxide.
Substitution: Primary amines and alcoholic potassium hydroxide.
Major Products Formed:
Oxidation: Phosgene, chlorine, and hydrogen chloride.
Hydrolysis: Potassium formate and deuterium chloride.
Substitution: Isocyanides.
Scientific Research Applications
Chloroform-d is extensively used in scientific research, particularly in the following fields:
Chemistry: As a solvent in NMR spectroscopy, this compound is used to dissolve a wide range of compounds and provide a clear NMR spectrum with minimal interference
Biology: It is used in the study of biological molecules and their interactions through NMR spectroscopy.
Medicine: this compound is used in the analysis of pharmaceutical compounds and their structures.
Industry: It is employed in the synthesis and analysis of various industrial chemicals and materials
Comparison with Similar Compounds
Chloroform (CHCl₃): The non-deuterated form of chloroform.
Deuterated Dichloromethane (CD₂Cl₂): Another deuterated solvent used in NMR spectroscopy.
Uniqueness of Chloroform-d: this compound is unique due to its cost-effectiveness, ease of production, and minimal interference in NMR spectroscopy. Its properties make it a preferred choice for many researchers in various fields .
Properties
IUPAC Name |
trichloro(deuterio)methane | |
---|---|---|
Source | PubChem | |
URL | https://pubchem.ncbi.nlm.nih.gov | |
Description | Data deposited in or computed by PubChem | |
InChI |
InChI=1S/CHCl3/c2-1(3)4/h1H/i1D | |
Source | PubChem | |
URL | https://pubchem.ncbi.nlm.nih.gov | |
Description | Data deposited in or computed by PubChem | |
InChI Key |
HEDRZPFGACZZDS-MICDWDOJSA-N | |
Source | PubChem | |
URL | https://pubchem.ncbi.nlm.nih.gov | |
Description | Data deposited in or computed by PubChem | |
Canonical SMILES |
C(Cl)(Cl)Cl | |
Source | PubChem | |
URL | https://pubchem.ncbi.nlm.nih.gov | |
Description | Data deposited in or computed by PubChem | |
Isomeric SMILES |
[2H]C(Cl)(Cl)Cl | |
Source | PubChem | |
URL | https://pubchem.ncbi.nlm.nih.gov | |
Description | Data deposited in or computed by PubChem | |
Molecular Formula |
CHCl3 | |
Source | PubChem | |
URL | https://pubchem.ncbi.nlm.nih.gov | |
Description | Data deposited in or computed by PubChem | |
DSSTOX Substance ID |
DTXSID50904766 | |
Record name | Deuterochloroform | |
Source | EPA DSSTox | |
URL | https://comptox.epa.gov/dashboard/DTXSID50904766 | |
Description | DSSTox provides a high quality public chemistry resource for supporting improved predictive toxicology. | |
Molecular Weight |
120.38 g/mol | |
Source | PubChem | |
URL | https://pubchem.ncbi.nlm.nih.gov | |
Description | Data deposited in or computed by PubChem | |
Physical Description |
Hygroscopic liquid; [Sigma-Aldrich MSDS] | |
Record name | Chloroform-d | |
Source | Haz-Map, Information on Hazardous Chemicals and Occupational Diseases | |
URL | https://haz-map.com/Agents/13652 | |
Description | Haz-Map® is an occupational health database designed for health and safety professionals and for consumers seeking information about the adverse effects of workplace exposures to chemical and biological agents. | |
Explanation | Copyright (c) 2022 Haz-Map(R). All rights reserved. Unless otherwise indicated, all materials from Haz-Map are copyrighted by Haz-Map(R). No part of these materials, either text or image may be used for any purpose other than for personal use. Therefore, reproduction, modification, storage in a retrieval system or retransmission, in any form or by any means, electronic, mechanical or otherwise, for reasons other than personal use, is strictly prohibited without prior written permission. | |
CAS No. |
865-49-6 | |
Record name | Chloroform-d | |
Source | CAS Common Chemistry | |
URL | https://commonchemistry.cas.org/detail?cas_rn=865-49-6 | |
Description | CAS Common Chemistry is an open community resource for accessing chemical information. Nearly 500,000 chemical substances from CAS REGISTRY cover areas of community interest, including common and frequently regulated chemicals, and those relevant to high school and undergraduate chemistry classes. This chemical information, curated by our expert scientists, is provided in alignment with our mission as a division of the American Chemical Society. | |
Explanation | The data from CAS Common Chemistry is provided under a CC-BY-NC 4.0 license, unless otherwise stated. | |
Record name | Chloroform-d | |
Source | ChemIDplus | |
URL | https://pubchem.ncbi.nlm.nih.gov/substance/?source=chemidplus&sourceid=0000865496 | |
Description | ChemIDplus is a free, web search system that provides access to the structure and nomenclature authority files used for the identification of chemical substances cited in National Library of Medicine (NLM) databases, including the TOXNET system. | |
Record name | Deuterochloroform | |
Source | EPA DSSTox | |
URL | https://comptox.epa.gov/dashboard/DTXSID50904766 | |
Description | DSSTox provides a high quality public chemistry resource for supporting improved predictive toxicology. | |
Record name | (2H)chloroform | |
Source | European Chemicals Agency (ECHA) | |
URL | https://echa.europa.eu/substance-information/-/substanceinfo/100.011.585 | |
Description | The European Chemicals Agency (ECHA) is an agency of the European Union which is the driving force among regulatory authorities in implementing the EU's groundbreaking chemicals legislation for the benefit of human health and the environment as well as for innovation and competitiveness. | |
Explanation | Use of the information, documents and data from the ECHA website is subject to the terms and conditions of this Legal Notice, and subject to other binding limitations provided for under applicable law, the information, documents and data made available on the ECHA website may be reproduced, distributed and/or used, totally or in part, for non-commercial purposes provided that ECHA is acknowledged as the source: "Source: European Chemicals Agency, http://echa.europa.eu/". Such acknowledgement must be included in each copy of the material. ECHA permits and encourages organisations and individuals to create links to the ECHA website under the following cumulative conditions: Links can only be made to webpages that provide a link to the Legal Notice page. | |
Record name | CHLOROFORM-D | |
Source | FDA Global Substance Registration System (GSRS) | |
URL | https://gsrs.ncats.nih.gov/ginas/app/beta/substances/P1NW4885VT | |
Description | The FDA Global Substance Registration System (GSRS) enables the efficient and accurate exchange of information on what substances are in regulated products. Instead of relying on names, which vary across regulatory domains, countries, and regions, the GSRS knowledge base makes it possible for substances to be defined by standardized, scientific descriptions. | |
Explanation | Unless otherwise noted, the contents of the FDA website (www.fda.gov), both text and graphics, are not copyrighted. They are in the public domain and may be republished, reprinted and otherwise used freely by anyone without the need to obtain permission from FDA. Credit to the U.S. Food and Drug Administration as the source is appreciated but not required. | |
Retrosynthesis Analysis
AI-Powered Synthesis Planning: Our tool employs the Template_relevance Pistachio, Template_relevance Bkms_metabolic, Template_relevance Pistachio_ringbreaker, Template_relevance Reaxys, Template_relevance Reaxys_biocatalysis model, leveraging a vast database of chemical reactions to predict feasible synthetic routes.
One-Step Synthesis Focus: Specifically designed for one-step synthesis, it provides concise and direct routes for your target compounds, streamlining the synthesis process.
Accurate Predictions: Utilizing the extensive PISTACHIO, BKMS_METABOLIC, PISTACHIO_RINGBREAKER, REAXYS, REAXYS_BIOCATALYSIS database, our tool offers high-accuracy predictions, reflecting the latest in chemical research and data.
Strategy Settings
Precursor scoring | Relevance Heuristic |
---|---|
Min. plausibility | 0.01 |
Model | Template_relevance |
Template Set | Pistachio/Bkms_metabolic/Pistachio_ringbreaker/Reaxys/Reaxys_biocatalysis |
Top-N result to add to graph | 6 |
Feasible Synthetic Routes
Disclaimer and Information on In-Vitro Research Products
Please be aware that all articles and product information presented on BenchChem are intended solely for informational purposes. The products available for purchase on BenchChem are specifically designed for in-vitro studies, which are conducted outside of living organisms. In-vitro studies, derived from the Latin term "in glass," involve experiments performed in controlled laboratory settings using cells or tissues. It is important to note that these products are not categorized as medicines or drugs, and they have not received approval from the FDA for the prevention, treatment, or cure of any medical condition, ailment, or disease. We must emphasize that any form of bodily introduction of these products into humans or animals is strictly prohibited by law. It is essential to adhere to these guidelines to ensure compliance with legal and ethical standards in research and experimentation.