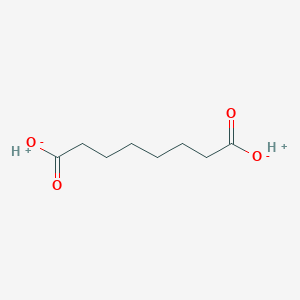
Suberic acid
Overview
Description
Suberic acid, also known as octanedioic acid, is a dicarboxylic acid with the chemical formula C₈H₁₄O₄. It is a colorless crystalline solid that is used in various industrial applications, including drug synthesis and plastics manufacture. The name “suberic” is derived from the Latin word “suber,” which means cork, as the compound was originally obtained from cork .
Mechanism of Action
Target of Action
Suberic acid, also known as octanedioic acid, is a dicarboxylic acid . It has been found to target the olfactory receptor OR10A3 . This receptor plays a crucial role in the detection of specific scents and is involved in various physiological processes .
Mode of Action
This compound interacts with its target, the olfactory receptor OR10A3, to promote collagen synthesis in ultraviolet B (UVB)-irradiated human dermal fibroblasts . This interaction helps to ameliorate UVB-induced decreases in collagen production , thereby playing a protective role against UVB radiation damage.
Biochemical Pathways
This compound is involved in the cAMP-Akt pathway . This pathway is crucial for various cellular processes, including cell growth, proliferation, differentiation, and survival . This compound’s activation of OR10A3 leads to an increase in collagen synthesis via this pathway . Additionally, this compound is a metabolic breakdown product derived from oleic acid .
Pharmacokinetics
It is known that this compound is present in the urine of patients with fatty acid oxidation disorders
Result of Action
The primary result of this compound’s action is the increased synthesis of collagen in UVB-irradiated human dermal fibroblasts . This leads to an amelioration of UVB-induced decreases in collagen production , which can help protect the skin from UVB radiation damage.
Action Environment
The action of this compound can be influenced by various environmental factors. For instance, UVB radiation can induce decreases in collagen production in dermal fibroblasts . This compound can counteract this effect by promoting collagen synthesis . .
Biochemical Analysis
Biochemical Properties
Suberic acid is involved in biochemical reactions, particularly in the context of fatty acid oxidation disorders . It is a metabolic breakdown product derived from oleic acid
Cellular Effects
This compound has been found to promote collagen synthesis in UVB-irradiated dermal fibroblasts via the cAMP-Akt pathway . This suggests that this compound can influence cell function, including impacts on cell signaling pathways and cellular metabolism .
Molecular Mechanism
It has been suggested that this compound can activate OR10A3, a specific olfactory receptor, which subsequently stimulates collagen synthesis via the downstream cAMP-Akt pathway .
Metabolic Pathways
This compound is a metabolic breakdown product derived from oleic acid . It is present in the urine of patients with fatty acid oxidation disorders
Preparation Methods
Synthetic Routes and Reaction Conditions
Suberic acid can be synthesized through the oxidation of cyclooctene using perrhenate-containing composite ionic liquids as green catalysts. This method involves the use of hydrogen peroxide as an oxidant, which is considered environmentally friendly . Another method involves the mechanochemical processing of betulin with this compound in the presence of small amounts of organic solvents such as ethanol, acetone, ethyl acetate, chloroform, toluene, and dioxane .
Industrial Production Methods
Industrial production of this compound typically involves the oxidation of castor oil, cyclic diols, or ketones in the presence of nitric acid as an oxidant. due to environmental concerns, alternative methods using aqueous hydrogen peroxide are being explored .
Chemical Reactions Analysis
Types of Reactions
Suberic acid undergoes various chemical reactions, including oxidation, reduction, and substitution. It acts as a Bronsted acid, capable of donating a proton to a Bronsted base .
Common Reagents and Conditions
Reduction: this compound can be reduced to its corresponding alcohols using reducing agents such as lithium aluminum hydride.
Substitution: It can undergo esterification reactions with alcohols in the presence of acid catalysts to form esters.
Major Products Formed
Oxidation: The oxidation of cyclooctene leads to the formation of this compound.
Reduction: Reduction of this compound yields octanediol.
Substitution: Esterification of this compound with methanol produces dimethyl suberate.
Scientific Research Applications
Suberic acid has a wide range of scientific research applications:
Comparison with Similar Compounds
Suberic acid is similar to other dicarboxylic acids such as azelaic acid, sebacic acid, and adipic acid. it has unique properties that distinguish it from these compounds:
Sebacic Acid: Sebacic acid (C₁₀H₁₈O₄) has a longer carbon chain and is used in the production of nylon and other polymers.
Adipic Acid: Adipic acid (C₆H₁₀O₄) has a shorter carbon chain and is widely used in the production of nylon-6,6.
This compound’s unique chain length and functional properties make it valuable in specific industrial and research applications.
Biological Activity
Suberic acid, a dicarboxylic acid with the chemical formula C8H14O4, is gaining attention for its potential biological activities, particularly in dermatological applications. This article reviews recent findings on the biological activity of this compound, focusing on its effects on collagen synthesis, anti-photoaging properties, and underlying molecular mechanisms.
- Molecular Formula : C8H14O4
- Structure : this compound is a colorless crystalline compound with two carboxylic acid functional groups.
1. Collagen Synthesis Promotion
Recent studies have demonstrated that this compound can enhance collagen synthesis in human dermal fibroblasts. A notable study revealed that this compound activates the olfactory receptor OR10A3, leading to increased collagen production in UVB-irradiated Hs68 cells. The activation of this receptor stimulates the cAMP-Akt signaling pathway, which is crucial for collagen synthesis.
- Key Findings :
2. Anti-photoaging Effects
This compound has been shown to protect against UVB-induced skin photoaging. In a study involving hairless mice exposed to UVB radiation, dietary supplementation with this compound significantly reduced skin dryness, wrinkle formation, and epidermal thickness.
- Study Design :
3. Molecular Mechanisms
The protective effects of this compound against UVB-induced damage are attributed to its modulation of various signaling pathways:
- Transforming Growth Factor-beta (TGF-β) Pathway : this compound upregulates TGF-β signaling, promoting collagen synthesis while inhibiting matrix metalloproteinases (MMPs) that degrade extracellular matrix components .
- Mitogen-Activated Protein Kinase (MAPK) Pathway : It downregulates MAPK pathway molecules involved in inflammation and cellular stress responses .
Table 1: Effects of this compound on Collagen Production
Concentration (µM) | Procollagen Production (Relative Units) | Cytotoxicity Observed |
---|---|---|
0 | Baseline | No |
50 | Increased | No |
100 | Saturated Increase | No |
200 | Slight Decrease | No |
400 | No Significant Change | No |
Table 2: Impact of Dietary this compound on Skin Parameters in Mice
Concentration (%) | Wrinkle Formation Reduction (%) | Epidermal Thickness Reduction (%) | Skin Dryness Score Reduction |
---|---|---|---|
0.05 | 20 | 15 | Moderate |
0.1 | 35 | 25 | Significant |
0.2 | 50 | 40 | High |
Case Studies
Several case studies highlight the effectiveness of this compound in mitigating skin aging:
- Case Study A : In a controlled trial with hairless mice, those receiving this compound showed a marked decrease in wrinkle depth and density compared to the control group subjected to UVB without treatment.
- Case Study B : Human dermal fibroblasts treated with this compound exhibited increased collagen I and III production, supporting its potential use in cosmetic formulations aimed at skin rejuvenation.
Properties
IUPAC Name |
octanedioic acid | |
---|---|---|
Source | PubChem | |
URL | https://pubchem.ncbi.nlm.nih.gov | |
Description | Data deposited in or computed by PubChem | |
InChI |
InChI=1S/C8H14O4/c9-7(10)5-3-1-2-4-6-8(11)12/h1-6H2,(H,9,10)(H,11,12) | |
Source | PubChem | |
URL | https://pubchem.ncbi.nlm.nih.gov | |
Description | Data deposited in or computed by PubChem | |
InChI Key |
TYFQFVWCELRYAO-UHFFFAOYSA-N | |
Source | PubChem | |
URL | https://pubchem.ncbi.nlm.nih.gov | |
Description | Data deposited in or computed by PubChem | |
Canonical SMILES |
C(CCCC(=O)O)CCC(=O)O | |
Source | PubChem | |
URL | https://pubchem.ncbi.nlm.nih.gov | |
Description | Data deposited in or computed by PubChem | |
Molecular Formula |
C8H14O4 | |
Source | PubChem | |
URL | https://pubchem.ncbi.nlm.nih.gov | |
Description | Data deposited in or computed by PubChem | |
DSSTOX Substance ID |
DTXSID8021644 | |
Record name | Octanedioic acid | |
Source | EPA DSSTox | |
URL | https://comptox.epa.gov/dashboard/DTXSID8021644 | |
Description | DSSTox provides a high quality public chemistry resource for supporting improved predictive toxicology. | |
Molecular Weight |
174.19 g/mol | |
Source | PubChem | |
URL | https://pubchem.ncbi.nlm.nih.gov | |
Description | Data deposited in or computed by PubChem | |
Physical Description |
Other Solid, Solid | |
Record name | Octanedioic acid | |
Source | EPA Chemicals under the TSCA | |
URL | https://www.epa.gov/chemicals-under-tsca | |
Description | EPA Chemicals under the Toxic Substances Control Act (TSCA) collection contains information on chemicals and their regulations under TSCA, including non-confidential content from the TSCA Chemical Substance Inventory and Chemical Data Reporting. | |
Record name | Suberic acid | |
Source | Human Metabolome Database (HMDB) | |
URL | http://www.hmdb.ca/metabolites/HMDB0000893 | |
Description | The Human Metabolome Database (HMDB) is a freely available electronic database containing detailed information about small molecule metabolites found in the human body. | |
Explanation | HMDB is offered to the public as a freely available resource. Use and re-distribution of the data, in whole or in part, for commercial purposes requires explicit permission of the authors and explicit acknowledgment of the source material (HMDB) and the original publication (see the HMDB citing page). We ask that users who download significant portions of the database cite the HMDB paper in any resulting publications. | |
Boiling Point |
345.50 °C. @ 760.00 mm Hg | |
Record name | Suberic acid | |
Source | Human Metabolome Database (HMDB) | |
URL | http://www.hmdb.ca/metabolites/HMDB0000893 | |
Description | The Human Metabolome Database (HMDB) is a freely available electronic database containing detailed information about small molecule metabolites found in the human body. | |
Explanation | HMDB is offered to the public as a freely available resource. Use and re-distribution of the data, in whole or in part, for commercial purposes requires explicit permission of the authors and explicit acknowledgment of the source material (HMDB) and the original publication (see the HMDB citing page). We ask that users who download significant portions of the database cite the HMDB paper in any resulting publications. | |
Solubility |
11.9 mg/mL | |
Record name | Suberic acid | |
Source | Human Metabolome Database (HMDB) | |
URL | http://www.hmdb.ca/metabolites/HMDB0000893 | |
Description | The Human Metabolome Database (HMDB) is a freely available electronic database containing detailed information about small molecule metabolites found in the human body. | |
Explanation | HMDB is offered to the public as a freely available resource. Use and re-distribution of the data, in whole or in part, for commercial purposes requires explicit permission of the authors and explicit acknowledgment of the source material (HMDB) and the original publication (see the HMDB citing page). We ask that users who download significant portions of the database cite the HMDB paper in any resulting publications. | |
CAS No. |
505-48-6, 68937-72-4 | |
Record name | Suberic acid | |
Source | CAS Common Chemistry | |
URL | https://commonchemistry.cas.org/detail?cas_rn=505-48-6 | |
Description | CAS Common Chemistry is an open community resource for accessing chemical information. Nearly 500,000 chemical substances from CAS REGISTRY cover areas of community interest, including common and frequently regulated chemicals, and those relevant to high school and undergraduate chemistry classes. This chemical information, curated by our expert scientists, is provided in alignment with our mission as a division of the American Chemical Society. | |
Explanation | The data from CAS Common Chemistry is provided under a CC-BY-NC 4.0 license, unless otherwise stated. | |
Record name | Suberic acid | |
Source | ChemIDplus | |
URL | https://pubchem.ncbi.nlm.nih.gov/substance/?source=chemidplus&sourceid=0000505486 | |
Description | ChemIDplus is a free, web search system that provides access to the structure and nomenclature authority files used for the identification of chemical substances cited in National Library of Medicine (NLM) databases, including the TOXNET system. | |
Record name | Carboxylic acids, di-, C4-11 | |
Source | ChemIDplus | |
URL | https://pubchem.ncbi.nlm.nih.gov/substance/?source=chemidplus&sourceid=0068937724 | |
Description | ChemIDplus is a free, web search system that provides access to the structure and nomenclature authority files used for the identification of chemical substances cited in National Library of Medicine (NLM) databases, including the TOXNET system. | |
Record name | Octanedioic acid | |
Source | DTP/NCI | |
URL | https://dtp.cancer.gov/dtpstandard/servlet/dwindex?searchtype=NSC&outputformat=html&searchlist=53777 | |
Description | The NCI Development Therapeutics Program (DTP) provides services and resources to the academic and private-sector research communities worldwide to facilitate the discovery and development of new cancer therapeutic agents. | |
Explanation | Unless otherwise indicated, all text within NCI products is free of copyright and may be reused without our permission. Credit the National Cancer Institute as the source. | |
Record name | Octanedioic acid | |
Source | DTP/NCI | |
URL | https://dtp.cancer.gov/dtpstandard/servlet/dwindex?searchtype=NSC&outputformat=html&searchlist=25952 | |
Description | The NCI Development Therapeutics Program (DTP) provides services and resources to the academic and private-sector research communities worldwide to facilitate the discovery and development of new cancer therapeutic agents. | |
Explanation | Unless otherwise indicated, all text within NCI products is free of copyright and may be reused without our permission. Credit the National Cancer Institute as the source. | |
Record name | Octanedioic acid | |
Source | EPA Chemicals under the TSCA | |
URL | https://www.epa.gov/chemicals-under-tsca | |
Description | EPA Chemicals under the Toxic Substances Control Act (TSCA) collection contains information on chemicals and their regulations under TSCA, including non-confidential content from the TSCA Chemical Substance Inventory and Chemical Data Reporting. | |
Record name | Octanedioic acid | |
Source | EPA DSSTox | |
URL | https://comptox.epa.gov/dashboard/DTXSID8021644 | |
Description | DSSTox provides a high quality public chemistry resource for supporting improved predictive toxicology. | |
Record name | Suberic acid | |
Source | European Chemicals Agency (ECHA) | |
URL | https://echa.europa.eu/substance-information/-/substanceinfo/100.007.283 | |
Description | The European Chemicals Agency (ECHA) is an agency of the European Union which is the driving force among regulatory authorities in implementing the EU's groundbreaking chemicals legislation for the benefit of human health and the environment as well as for innovation and competitiveness. | |
Explanation | Use of the information, documents and data from the ECHA website is subject to the terms and conditions of this Legal Notice, and subject to other binding limitations provided for under applicable law, the information, documents and data made available on the ECHA website may be reproduced, distributed and/or used, totally or in part, for non-commercial purposes provided that ECHA is acknowledged as the source: "Source: European Chemicals Agency, http://echa.europa.eu/". Such acknowledgement must be included in each copy of the material. ECHA permits and encourages organisations and individuals to create links to the ECHA website under the following cumulative conditions: Links can only be made to webpages that provide a link to the Legal Notice page. | |
Record name | Carboxylic acids, di-, C4-11 | |
Source | European Chemicals Agency (ECHA) | |
URL | https://echa.europa.eu/substance-information/-/substanceinfo/100.066.420 | |
Description | The European Chemicals Agency (ECHA) is an agency of the European Union which is the driving force among regulatory authorities in implementing the EU's groundbreaking chemicals legislation for the benefit of human health and the environment as well as for innovation and competitiveness. | |
Explanation | Use of the information, documents and data from the ECHA website is subject to the terms and conditions of this Legal Notice, and subject to other binding limitations provided for under applicable law, the information, documents and data made available on the ECHA website may be reproduced, distributed and/or used, totally or in part, for non-commercial purposes provided that ECHA is acknowledged as the source: "Source: European Chemicals Agency, http://echa.europa.eu/". Such acknowledgement must be included in each copy of the material. ECHA permits and encourages organisations and individuals to create links to the ECHA website under the following cumulative conditions: Links can only be made to webpages that provide a link to the Legal Notice page. | |
Record name | SUBERIC ACID | |
Source | FDA Global Substance Registration System (GSRS) | |
URL | https://gsrs.ncats.nih.gov/ginas/app/beta/substances/6U7Y4M9C1H | |
Description | The FDA Global Substance Registration System (GSRS) enables the efficient and accurate exchange of information on what substances are in regulated products. Instead of relying on names, which vary across regulatory domains, countries, and regions, the GSRS knowledge base makes it possible for substances to be defined by standardized, scientific descriptions. | |
Explanation | Unless otherwise noted, the contents of the FDA website (www.fda.gov), both text and graphics, are not copyrighted. They are in the public domain and may be republished, reprinted and otherwise used freely by anyone without the need to obtain permission from FDA. Credit to the U.S. Food and Drug Administration as the source is appreciated but not required. | |
Record name | Suberic acid | |
Source | Human Metabolome Database (HMDB) | |
URL | http://www.hmdb.ca/metabolites/HMDB0000893 | |
Description | The Human Metabolome Database (HMDB) is a freely available electronic database containing detailed information about small molecule metabolites found in the human body. | |
Explanation | HMDB is offered to the public as a freely available resource. Use and re-distribution of the data, in whole or in part, for commercial purposes requires explicit permission of the authors and explicit acknowledgment of the source material (HMDB) and the original publication (see the HMDB citing page). We ask that users who download significant portions of the database cite the HMDB paper in any resulting publications. | |
Melting Point |
144 °C | |
Record name | Suberic acid | |
Source | Human Metabolome Database (HMDB) | |
URL | http://www.hmdb.ca/metabolites/HMDB0000893 | |
Description | The Human Metabolome Database (HMDB) is a freely available electronic database containing detailed information about small molecule metabolites found in the human body. | |
Explanation | HMDB is offered to the public as a freely available resource. Use and re-distribution of the data, in whole or in part, for commercial purposes requires explicit permission of the authors and explicit acknowledgment of the source material (HMDB) and the original publication (see the HMDB citing page). We ask that users who download significant portions of the database cite the HMDB paper in any resulting publications. | |
Retrosynthesis Analysis
AI-Powered Synthesis Planning: Our tool employs the Template_relevance Pistachio, Template_relevance Bkms_metabolic, Template_relevance Pistachio_ringbreaker, Template_relevance Reaxys, Template_relevance Reaxys_biocatalysis model, leveraging a vast database of chemical reactions to predict feasible synthetic routes.
One-Step Synthesis Focus: Specifically designed for one-step synthesis, it provides concise and direct routes for your target compounds, streamlining the synthesis process.
Accurate Predictions: Utilizing the extensive PISTACHIO, BKMS_METABOLIC, PISTACHIO_RINGBREAKER, REAXYS, REAXYS_BIOCATALYSIS database, our tool offers high-accuracy predictions, reflecting the latest in chemical research and data.
Strategy Settings
Precursor scoring | Relevance Heuristic |
---|---|
Min. plausibility | 0.01 |
Model | Template_relevance |
Template Set | Pistachio/Bkms_metabolic/Pistachio_ringbreaker/Reaxys/Reaxys_biocatalysis |
Top-N result to add to graph | 6 |
Feasible Synthetic Routes
Disclaimer and Information on In-Vitro Research Products
Please be aware that all articles and product information presented on BenchChem are intended solely for informational purposes. The products available for purchase on BenchChem are specifically designed for in-vitro studies, which are conducted outside of living organisms. In-vitro studies, derived from the Latin term "in glass," involve experiments performed in controlled laboratory settings using cells or tissues. It is important to note that these products are not categorized as medicines or drugs, and they have not received approval from the FDA for the prevention, treatment, or cure of any medical condition, ailment, or disease. We must emphasize that any form of bodily introduction of these products into humans or animals is strictly prohibited by law. It is essential to adhere to these guidelines to ensure compliance with legal and ethical standards in research and experimentation.