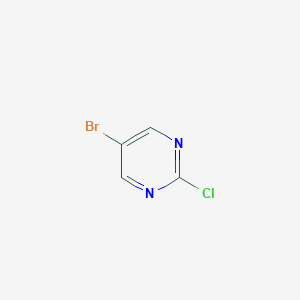
5-Bromo-2-chloropyrimidine
Overview
Description
Chemical Identity:
5-Bromo-2-chloropyrimidine (CAS: 32779-36-5) is a halogenated heterocyclic compound with the molecular formula C₄H₂BrClN₂ and a molecular weight of 193.43 g/mol . It is a white to beige crystalline powder with a melting point of 78–80°C and is commercially available in purities ranging from 95% to 97% .
Applications:
This compound serves as a versatile intermediate in organic synthesis, particularly in:
- Cross-coupling reactions (e.g., Ni- or Pd-catalyzed couplings) for constructing C–C bonds .
- Pharmaceutical synthesis, such as in the development of endothelin receptor antagonists (e.g., pipersentan) .
- Material science, including the synthesis of thermally activated delayed fluorescence (TADF) emitters for OLEDs .
Preparation Methods
Synthetic Routes and Reaction Conditions: One common method for synthesizing 5-Bromo-2-chloropyrimidine involves the reaction of 2-hydroxy-5-bromopyrimidine with phosphorus oxychloride in the presence of an organic amine such as triethylamine. The reaction is typically carried out under nitrogen protection at a temperature of 80-85°C for about 6 hours. After the reaction, the mixture is cooled, and the product is purified .
Industrial Production Methods: In industrial settings, the synthesis of this compound can be optimized by using a one-step method. This involves heating 2-hydroxypyrimidine with hydrobromic acid and hydrogen peroxide, followed by a reaction with phosphorus oxychloride and an organic amine. This method significantly improves production efficiency and yield .
Chemical Reactions Analysis
Cross-Coupling Reactions
5-Bromo-2-chloropyrimidine serves as a key electrophilic partner in transition-metal-catalyzed cross-coupling reactions. The bromine atom at position 5 is more reactive than the chlorine at position 2, allowing selective functionalization.
Examples of Cross-Coupling Reactions
- Mechanistic Insight : The bromine atom undergoes oxidative addition with palladium(0) catalysts, forming a Pd(II) intermediate. Transmetallation with organoindium reagents followed by reductive elimination yields the coupled product .
- Selectivity : The chlorine at position 2 remains intact under these conditions, enabling sequential functionalization .
Nucleophilic Substitution Reactions
The 2-chloro group in this compound is susceptible to nucleophilic displacement, particularly under basic or catalytic conditions.
Key Substitution Pathways
- Reactivity Trends : The electron-withdrawing bromine at position 5 enhances the electrophilicity of the adjacent chlorine, facilitating substitution .
- Catalytic Systems : Copper iodide (CuI) accelerates amination reactions by stabilizing intermediates .
Sequential Functionalization Strategies
The differential reactivity of the 2-chloro and 5-bromo positions allows for stepwise modifications:
- Cross-Coupling at C5 :
- Substitution at C2 :
Stability and Handling Considerations
Scientific Research Applications
Chemistry: 5-Bromo-2-chloropyrimidine is used as a building block in the synthesis of more complex heterocyclic compounds. It is particularly valuable in the development of pharmaceuticals and agrochemicals .
Biology and Medicine: In medicinal chemistry, this compound serves as an intermediate in the synthesis of various inhibitors and other bioactive molecules. It is used in the development of drugs targeting specific enzymes and receptors .
Industry: In the agrochemical industry, this compound is used in the synthesis of pesticides and herbicides. Its unique reactivity makes it a valuable intermediate in the production of various agrochemical products .
Mechanism of Action
The mechanism of action of 5-Bromo-2-chloropyrimidine is primarily based on its ability to undergo nucleophilic substitution and cross-coupling reactions. The chlorine and bromine atoms in its structure make it highly reactive towards nucleophiles and organometallic reagents. This reactivity allows it to form various substituted pyrimidines, which can interact with biological targets such as enzymes and receptors .
Comparison with Similar Compounds
Comparison with Structurally Similar Compounds
The following table summarizes key derivatives and analogs of 5-bromo-2-chloropyrimidine, highlighting structural and functional differences:
Reactivity and Selectivity
- Halogen Reactivity : In this compound, the bromine atom at C5 is more reactive than chlorine in cross-coupling reactions due to lower bond dissociation energy. For example, Ni-catalyzed couplings selectively substitute bromine while retaining chlorine . In contrast, 5-bromo-2,4-dichloropyrimidine allows sequential substitution at C5 (Br) followed by C2/C4 (Cl), enabling modular synthesis .
- Amino Substitution: Introducing an NH₂ group at C4 (as in 4-amino-5-bromo-2-chloropyrimidine) enhances hydrogen bonding, as observed in its crystal structure, forming 2D networks via N–H···N interactions . This property is absent in the parent compound.
Physical and Electronic Properties
- Solubility: The amino derivative exhibits slight water solubility due to hydrogen-bonding capability, whereas this compound is hydrophobic .
- Electronic Effects : Photoelectron spectroscopy reveals that the HOMO of this compound is localized on the pyrimidine ring and halogen lone pairs, with ionization energy at 11.9 eV . Derivatives with electron-donating groups (e.g., NH₂) may shift these energy levels, influencing optoelectronic applications .
Biological Activity
5-Bromo-2-chloropyrimidine is a heterocyclic compound that has garnered attention for its diverse biological activities, particularly in the fields of medicinal chemistry and pharmacology. This article provides a comprehensive overview of the biological activity associated with this compound, highlighting its potential therapeutic applications, mechanisms of action, and relevant research findings.
Chemical Structure and Properties
This compound is characterized by a pyrimidine ring substituted with bromine and chlorine atoms. The presence of these halogens contributes to its reactivity and biological properties. Its chemical structure can be represented as follows:
Anticancer Activity
Numerous studies have explored the anticancer properties of this compound and its derivatives. A notable study synthesized various bromo-pyrimidine derivatives, including this compound, and evaluated their cytotoxic effects against several tumor cell lines, such as HCT116 (colon cancer), A549 (lung cancer), and K562 (chronic myeloid leukemia) using the MTT assay. The results indicated that certain derivatives exhibited potent inhibitory activity against Bcr/Abl tyrosine kinase, a target in cancer therapy, suggesting their potential as alternative treatments to existing therapies like Dasatinib .
Table 1: Cytotoxic Activity of this compound Derivatives
Compound | Cell Line | IC50 (µM) | Mechanism of Action |
---|---|---|---|
5b | K562 | 1.2 | Bcr/Abl Inhibition |
5c | A549 | 2.3 | Apoptosis Induction |
5e | HCT116 | 0.9 | Cell Cycle Arrest |
Anticonvulsant Activity
Research has also indicated that derivatives of this compound possess anticonvulsant properties. A study focused on synthesizing 1-(5-bromo-2-chloropyrimidin-4-yl)hydrazine derivatives demonstrated significant anticonvulsant activity in animal models. The mechanism is believed to involve modulation of neurotransmitter systems, although specific pathways remain to be fully elucidated .
Antimicrobial Properties
The compound has shown promise in antimicrobial applications as well. Various studies have reported that pyrimidine derivatives exhibit broad-spectrum antimicrobial activity against both bacterial and fungal strains. This activity is attributed to the ability of these compounds to interfere with microbial DNA synthesis or function .
The biological activities of this compound can be attributed to several mechanisms:
- Kinase Inhibition : The compound acts as a potent inhibitor of Bcr/Abl kinase, which is crucial in the treatment of certain leukemias.
- DNA Intercalation : Some studies suggest that it may intercalate into DNA, disrupting replication processes.
- Neurotransmitter Modulation : In anticonvulsant applications, it may influence neurotransmitter levels or receptor activity.
Case Studies
- Bcr/Abl Kinase Inhibition : In a study evaluating various bromo-pyrimidine derivatives, compounds were tested against K562 cells. The most effective inhibitors showed IC50 values significantly lower than Dasatinib, indicating their potential as effective alternatives in targeted cancer therapies .
- Anticonvulsant Efficacy : A series of experiments conducted on animal models demonstrated that hydrazine derivatives of this compound significantly reduced seizure frequency and duration compared to control groups, suggesting a strong anticonvulsant profile .
Q & A
Q. What are the common synthetic routes for preparing 5-bromo-2-chloropyrimidine, and what factors influence yield and purity?
Basic
this compound is typically synthesized via chlorination of 5-bromo-2-hydroxypyrimidine using phosphorus oxychloride (POCl₃) under reflux, achieving yields of ~70% (). Alternatively, 5-bromo-2-methoxypyrimidine can be converted to the chloro derivative using similar methods, with yields up to 95% (). Reaction conditions, such as temperature (50–110°C) and stoichiometry of chlorinating agents, significantly impact purity. Post-synthesis purification via recrystallization (e.g., from acetonitrile) ensures >95% purity ().
Q. How can the electronic structure of this compound be analyzed experimentally and computationally?
Basic
The electronic structure is characterized using He I photoelectron spectroscopy (PES) , which identifies ionization energies (e.g., the first ionization band at 11.9 eV corresponds to π₂ orbitals and halogen lone pairs) (). Computational methods like density functional theory (DFT) with gradient-corrected exchange-correlation functionals (e.g., B3LYP) model molecular orbitals (MOs) and validate experimental data ( ). These studies reveal contributions from bromine (nBr) and chlorine (nCl) lone pairs to the HOMO and LUMO, guiding reactivity predictions.
Q. What challenges arise in achieving regioselective cross-coupling reactions with this compound?
Advanced
Regioselectivity is influenced by the differing reactivity of bromine (C5) and chlorine (C2) substituents. Palladium-catalyzed cross-coupling with triorganoindium reagents (R₃In) selectively substitutes bromine first at 40 mol% R₃In, yielding 5-substituted-2-chloropyrimidines. At 100 mol% R₃In, sequential substitution occurs, forming 2,5-disubstituted pyrimidines ( ). Ligand choice (e.g., bidentate ligands for pathway B) and leaving group nucleophilicity (e.g., triflate vs. chloride) further modulate selectivity ().
Q. How do computational methods aid in understanding the reactivity and stability of this compound derivatives?
Advanced
DFT calculations with exact-exchange functionals (e.g., B3LYP) predict activation barriers for substitution reactions and compare favorably with experimental kinetic data ( ). For example, computational studies on hydrogen-bonding networks in derivatives (e.g., 5-bromo-2-chloropyrimidin-4-amine) reveal stabilization via N–H···N interactions, explaining crystallographic planar arrangements (r.m.s. deviation: 0.087 Å) (). These insights guide solvent selection and catalyst design for stabilizing intermediates.
Q. What spectroscopic and crystallographic techniques are critical for characterizing this compound?
Basic
- Infrared (IR) spectroscopy : Confirms functional groups (e.g., C–Br stretch at ~550 cm⁻¹) and absence of hydroxyl impurities.
- X-ray crystallography : Resolves the planar pyrimidine ring (planarity deviation: 0.087 Å) and hydrogen-bonded dimers (N7–H···N3/N1 interactions) ().
- NMR : ¹H/¹³C spectra identify substituent positions (e.g., deshielded C5 due to bromine’s electron-withdrawing effect).
Q. How does steric and electronic effects influence the chemoselectivity of nucleophilic substitutions in this compound?
Advanced
Bromine’s lower electronegativity (vs. chlorine) and larger atomic radius make C5 more susceptible to nucleophilic attack. In Suzuki-Miyaura couplings, bromine is preferentially substituted under mild conditions (e.g., Pd(PPh₃)₄, 80°C), while chlorine requires harsher catalysts (e.g., XPhos Pd G3) ( ). Steric hindrance at C2 (due to chlorine’s proximity to the ring) further disfavors substitution at this position unless activated by electron-donating groups ().
Q. What are the thermodynamic considerations in synthesizing this compound derivatives?
Advanced
Reaction pathways (e.g., SNAr vs. oxidative addition) are governed by thermodynamic stability. For example, the conversion of 5-bromo-2-chloro-4-nitropyrimidine to the amine derivative (via SnCl₂ reduction) is exothermic (ΔH = −28 kcal/mol), favoring high yields (90%) (). Solvent polarity (e.g., DMF for polar transition states) and temperature (reflux at 110°C for dioxane-based reactions) optimize activation energy barriers ( ).
Q. How do reaction conditions affect the scalability of this compound synthesis?
Advanced
Scalability requires balancing reaction time, catalyst loading, and purification. For instance, one-pot sequential cross-couplings (e.g., Pd-catalyzed with R₃In) reduce intermediate isolation steps, achieving 80% yield for multigram syntheses ( ). Recrystallization from ethanol or acetonitrile ensures minimal loss (<5%) at scale (). Catalyst recycling (e.g., Pd nanoparticles) and solvent recovery (e.g., dioxane) improve sustainability.
Q. What are the key differences in the reaction mechanisms of this compound compared to other dihalopyrimidines?
Advanced
The Br/Cl pair in this compound exhibits distinct electronic effects vs. dihalides like 2,5-dibromopyrimidine. Bromine’s lower electronegativity increases electron density at C5, accelerating oxidative addition in Pd-catalyzed reactions ( ). In contrast, 2,5-dichloropyrimidine requires stronger bases (e.g., NaOtBu) for activation. Computational studies ( ) correlate these trends with MO energies, where bromine’s nBr orbital lowers the LUMO at C5.
Q. How can hydrogen-bonding interactions in this compound derivatives be exploited in supramolecular chemistry?
Advanced
Derivatives like 5-bromo-2-chloropyrimidin-4-amine form 2D networks via N–H···N hydrogen bonds (2.8–3.0 Å), as shown in X-ray studies (). These interactions stabilize co-crystals with π-acceptors (e.g., TCNQ), enabling applications in organic semiconductors. Solvent polarity (e.g., acetonitrile vs. ethyl acetate) modulates crystal packing, as seen in differing melting points (78–80°C vs. 460–461 K for derivatives) ( ).
Properties
IUPAC Name |
5-bromo-2-chloropyrimidine | |
---|---|---|
Source | PubChem | |
URL | https://pubchem.ncbi.nlm.nih.gov | |
Description | Data deposited in or computed by PubChem | |
InChI |
InChI=1S/C4H2BrClN2/c5-3-1-7-4(6)8-2-3/h1-2H | |
Source | PubChem | |
URL | https://pubchem.ncbi.nlm.nih.gov | |
Description | Data deposited in or computed by PubChem | |
InChI Key |
XPGIBDJXEVAVTO-UHFFFAOYSA-N | |
Source | PubChem | |
URL | https://pubchem.ncbi.nlm.nih.gov | |
Description | Data deposited in or computed by PubChem | |
Canonical SMILES |
C1=C(C=NC(=N1)Cl)Br | |
Source | PubChem | |
URL | https://pubchem.ncbi.nlm.nih.gov | |
Description | Data deposited in or computed by PubChem | |
Molecular Formula |
C4H2BrClN2 | |
Record name | 5-bromo-2-chloropyrimidine | |
Source | Wikipedia | |
URL | https://en.wikipedia.org/wiki/Dictionary_of_chemical_formulas | |
Description | Chemical information link to Wikipedia. | |
Source | PubChem | |
URL | https://pubchem.ncbi.nlm.nih.gov | |
Description | Data deposited in or computed by PubChem | |
DSSTOX Substance ID |
DTXSID50345731 | |
Record name | 5-Bromo-2-chloropyrimidine | |
Source | EPA DSSTox | |
URL | https://comptox.epa.gov/dashboard/DTXSID50345731 | |
Description | DSSTox provides a high quality public chemistry resource for supporting improved predictive toxicology. | |
Molecular Weight |
193.43 g/mol | |
Source | PubChem | |
URL | https://pubchem.ncbi.nlm.nih.gov | |
Description | Data deposited in or computed by PubChem | |
CAS No. |
32779-36-5 | |
Record name | 5-Bromo-2-chloropyrimidine | |
Source | EPA DSSTox | |
URL | https://comptox.epa.gov/dashboard/DTXSID50345731 | |
Description | DSSTox provides a high quality public chemistry resource for supporting improved predictive toxicology. | |
Record name | 5-Bromo-2-chloropyrimidine | |
Source | European Chemicals Agency (ECHA) | |
URL | https://echa.europa.eu/information-on-chemicals | |
Description | The European Chemicals Agency (ECHA) is an agency of the European Union which is the driving force among regulatory authorities in implementing the EU's groundbreaking chemicals legislation for the benefit of human health and the environment as well as for innovation and competitiveness. | |
Explanation | Use of the information, documents and data from the ECHA website is subject to the terms and conditions of this Legal Notice, and subject to other binding limitations provided for under applicable law, the information, documents and data made available on the ECHA website may be reproduced, distributed and/or used, totally or in part, for non-commercial purposes provided that ECHA is acknowledged as the source: "Source: European Chemicals Agency, http://echa.europa.eu/". Such acknowledgement must be included in each copy of the material. ECHA permits and encourages organisations and individuals to create links to the ECHA website under the following cumulative conditions: Links can only be made to webpages that provide a link to the Legal Notice page. | |
Retrosynthesis Analysis
AI-Powered Synthesis Planning: Our tool employs the Template_relevance Pistachio, Template_relevance Bkms_metabolic, Template_relevance Pistachio_ringbreaker, Template_relevance Reaxys, Template_relevance Reaxys_biocatalysis model, leveraging a vast database of chemical reactions to predict feasible synthetic routes.
One-Step Synthesis Focus: Specifically designed for one-step synthesis, it provides concise and direct routes for your target compounds, streamlining the synthesis process.
Accurate Predictions: Utilizing the extensive PISTACHIO, BKMS_METABOLIC, PISTACHIO_RINGBREAKER, REAXYS, REAXYS_BIOCATALYSIS database, our tool offers high-accuracy predictions, reflecting the latest in chemical research and data.
Strategy Settings
Precursor scoring | Relevance Heuristic |
---|---|
Min. plausibility | 0.01 |
Model | Template_relevance |
Template Set | Pistachio/Bkms_metabolic/Pistachio_ringbreaker/Reaxys/Reaxys_biocatalysis |
Top-N result to add to graph | 6 |
Feasible Synthetic Routes
Disclaimer and Information on In-Vitro Research Products
Please be aware that all articles and product information presented on BenchChem are intended solely for informational purposes. The products available for purchase on BenchChem are specifically designed for in-vitro studies, which are conducted outside of living organisms. In-vitro studies, derived from the Latin term "in glass," involve experiments performed in controlled laboratory settings using cells or tissues. It is important to note that these products are not categorized as medicines or drugs, and they have not received approval from the FDA for the prevention, treatment, or cure of any medical condition, ailment, or disease. We must emphasize that any form of bodily introduction of these products into humans or animals is strictly prohibited by law. It is essential to adhere to these guidelines to ensure compliance with legal and ethical standards in research and experimentation.