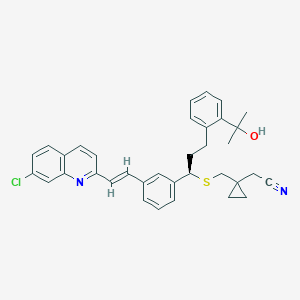
Montelukast nitrile
Overview
Description
Montelukast nitrile is a derivative of montelukast, a leukotriene receptor antagonist primarily used in the treatment of asthma and allergic rhinitis
Mechanism of Action
Target of Action
Montelukast nitrile primarily targets the leukotriene receptors in the body . Leukotrienes are inflammatory mediators produced by the body during an immune response . Montelukast is a leukotriene receptor antagonist , meaning it blocks the action of leukotrienes, thereby reducing inflammation and constriction in the airways .
Mode of Action
This compound works by selectively and effectively inhibiting the cysteinyl leukotriene receptor . By blocking the action of leukotriene D4 in the lungs, it results in decreased inflammation and relaxation of smooth muscle . This inhibition prevents the action of leukotrienes, which are substances in the body that cause narrowing and swelling of airways in the lungs .
Biochemical Pathways
The biochemical pathway affected by this compound is the 5-lipooxygenase (5-LO) pathway . Leukotrienes, the primary targets of montelukast, are products of this pathway. By blocking the leukotriene receptors, montelukast prevents the pro-inflammatory actions of leukotrienes, such as bronchoconstriction and increased endothelial permeability .
Pharmacokinetics
The pharmacokinetics of montelukast involve its absorption, distribution, metabolism, and excretion (ADME). Montelukast is administered orally and has a bioavailability of 63–73% . It is highly protein-bound (99%) and is metabolized in the liver primarily by the CYP2C8 enzyme, with minor contributions from the CYP3A4 and CYP2C9 enzymes . The elimination half-life of montelukast is between 2.7–5.5 hours, and it is excreted in the bile .
Result of Action
The molecular and cellular effects of montelukast’s action primarily involve the reduction of inflammation and relaxation of smooth muscle in the lungs . This results in the prevention of asthma symptoms, exercise-induced bronchoconstriction, and the treatment of seasonal allergic rhinitis .
Action Environment
The action, efficacy, and stability of montelukast can be influenced by various environmental factors. For instance, the presence of other medications can affect its metabolism and efficacy. Additionally, patient-specific factors such as genetic variations in the metabolizing enzymes can also influence the drug’s effectiveness
Biochemical Analysis
Biochemical Properties
Montelukast nitrile, like its parent compound Montelukast, is expected to interact with various biomolecules. It selectively and competitively blocks the cysteinyl leukotriene 1 (CysLT1) receptor, preventing the binding of the inflammatory mediator leukotriene D4 (LTD4) .
Cellular Effects
Montelukast, its parent compound, has been shown to influence cell function by blocking the CysLT1 receptor, which plays a role in cell signaling pathways, gene expression, and cellular metabolism .
Molecular Mechanism
It is likely to be similar to that of Montelukast, which exerts its effects at the molecular level by blocking the CysLT1 receptor, thereby preventing the binding of LTD4 .
Preparation Methods
Synthetic Routes and Reaction Conditions: The synthesis of montelukast nitrile typically involves the introduction of a nitrile group into the montelukast molecule. One common method is the reaction of montelukast with a nitrile-containing reagent under specific conditions. For example, the nitrile group can be introduced through a nucleophilic substitution reaction using a suitable nitrile reagent.
Industrial Production Methods: Industrial production of this compound may involve multi-step synthesis processes, including the preparation of intermediates and their subsequent conversion to the final product. The process often requires precise control of reaction conditions, such as temperature, pressure, and pH, to ensure high yield and purity.
Chemical Reactions Analysis
Types of Reactions: Montelukast nitrile can undergo various chemical reactions, including:
Oxidation: The nitrile group can be oxidized to form carboxylic acids or other derivatives.
Reduction: The nitrile group can be reduced to primary amines using reducing agents like lithium aluminum hydride.
Substitution: The nitrile group can participate in nucleophilic substitution reactions, leading to the formation of different derivatives.
Common Reagents and Conditions:
Oxidation: Reagents such as potassium permanganate or chromium trioxide can be used under acidic or basic conditions.
Reduction: Lithium aluminum hydride or catalytic hydrogenation can be employed under anhydrous conditions.
Substitution: Nucleophiles like amines or alcohols can react with the nitrile group under mild to moderate conditions.
Major Products:
Oxidation: Carboxylic acids or their derivatives.
Reduction: Primary amines.
Substitution: Various substituted derivatives depending on the nucleophile used.
Scientific Research Applications
Montelukast nitrile has several scientific research applications, including:
Chemistry: Used as a building block for the synthesis of more complex molecules.
Biology: Studied for its potential effects on biological systems, particularly in relation to leukotriene pathways.
Medicine: Investigated for its potential therapeutic effects, including anti-inflammatory and anti-asthmatic properties.
Industry: Utilized in the development of new pharmaceuticals and chemical products.
Comparison with Similar Compounds
Montelukast: The parent compound, primarily used for asthma and allergic rhinitis.
Zafirlukast: Another leukotriene receptor antagonist with similar therapeutic uses.
Pranlukast: A leukotriene receptor antagonist used in the treatment of asthma.
Uniqueness: Montelukast nitrile is unique due to the presence of the nitrile group, which can alter its chemical properties and potentially enhance its therapeutic effects. The nitrile group may also provide additional sites for chemical modification, leading to the development of new derivatives with improved efficacy and safety profiles.
Biological Activity
Montelukast nitrile, a derivative of montelukast, has garnered attention for its potential biological activities, particularly in the context of respiratory diseases and viral infections. This article reviews the biological activity of this compound, focusing on its mechanisms of action, efficacy in various applications, and safety profile based on recent studies.
Overview of this compound
Montelukast is primarily known as a cysteinyl-leukotriene receptor antagonist used in the treatment of asthma and allergic rhinitis. The nitrile form has been investigated for its enhanced pharmacological properties, including its antiviral effects against SARS-CoV-2 and anti-inflammatory activities.
1. Inhibition of Viral Activity
Recent studies have demonstrated that this compound exhibits significant antiviral activity against SARS-CoV-2. In vitro experiments showed that montelukast inhibits the main protease (Mpro) of the virus, crucial for viral replication. Key findings include:
- Mpro Inhibition : At a concentration of 100 µM, this compound resulted in a 74% reduction in Mpro activity. The IC50 value was determined to be approximately 28.36 µM, indicating a strong binding affinity to the enzyme .
- Binding Energy Calculations : Molecular dynamics simulations revealed an average binding free energy of -79.60 ± 8.66 kcal/mol at the Mpro site and -43.93 ± 7.66 kcal/mol at the spike/ACE2 interface, suggesting robust interactions that could impede viral entry into host cells .
2. Anti-inflammatory Properties
This compound also demonstrates anti-inflammatory effects by modulating immune responses:
- T Lymphocyte Modulation : Studies indicate that montelukast reduces T cell proliferation and increases apoptosis in allergen-specific T cells at low concentrations (10^-6 M). This effect is mediated through alterations in gene expression associated with apoptosis and inflammatory responses .
Case Study 1: Neuropsychiatric Adverse Events
A population-based case-crossover study assessed the risk of neuropsychiatric adverse events (NPAEs) associated with montelukast use in pediatric patients. The study found increased risks across various time windows after montelukast exposure, highlighting the need for cautious prescribing practices .
Hazard Period | Adjusted Odds Ratio (aOR) | 95% Confidence Interval |
---|---|---|
3 days | 1.28 | 1.24 to 1.32 |
7 days | 1.29 | 1.26 to 1.33 |
14 days | 1.34 | 1.31 to 1.37 |
28 days | 1.38 | 1.36 to 1.41 |
56 days | 1.21 | 1.19 to 1.22 |
This underscores the importance of monitoring for psychological side effects during treatment.
Efficacy Against Respiratory Viruses
The dual action of this compound as both an antiviral and anti-inflammatory agent makes it a candidate for further investigation in respiratory viral infections beyond COVID-19:
Properties
IUPAC Name |
2-[1-[[(1R)-1-[3-[(E)-2-(7-chloroquinolin-2-yl)ethenyl]phenyl]-3-[2-(2-hydroxypropan-2-yl)phenyl]propyl]sulfanylmethyl]cyclopropyl]acetonitrile | |
---|---|---|
Source | PubChem | |
URL | https://pubchem.ncbi.nlm.nih.gov | |
Description | Data deposited in or computed by PubChem | |
InChI |
InChI=1S/C35H35ClN2OS/c1-34(2,39)31-9-4-3-7-26(31)13-17-33(40-24-35(18-19-35)20-21-37)28-8-5-6-25(22-28)10-15-30-16-12-27-11-14-29(36)23-32(27)38-30/h3-12,14-16,22-23,33,39H,13,17-20,24H2,1-2H3/b15-10+/t33-/m1/s1 | |
Source | PubChem | |
URL | https://pubchem.ncbi.nlm.nih.gov | |
Description | Data deposited in or computed by PubChem | |
InChI Key |
LFNFSZSLPYLMBG-LDXVMNHOSA-N | |
Source | PubChem | |
URL | https://pubchem.ncbi.nlm.nih.gov | |
Description | Data deposited in or computed by PubChem | |
Canonical SMILES |
CC(C)(C1=CC=CC=C1CCC(C2=CC=CC(=C2)C=CC3=NC4=C(C=CC(=C4)Cl)C=C3)SCC5(CC5)CC#N)O | |
Source | PubChem | |
URL | https://pubchem.ncbi.nlm.nih.gov | |
Description | Data deposited in or computed by PubChem | |
Isomeric SMILES |
CC(C)(C1=CC=CC=C1CC[C@H](C2=CC=CC(=C2)/C=C/C3=NC4=C(C=CC(=C4)Cl)C=C3)SCC5(CC5)CC#N)O | |
Source | PubChem | |
URL | https://pubchem.ncbi.nlm.nih.gov | |
Description | Data deposited in or computed by PubChem | |
Molecular Formula |
C35H35ClN2OS | |
Source | PubChem | |
URL | https://pubchem.ncbi.nlm.nih.gov | |
Description | Data deposited in or computed by PubChem | |
Molecular Weight |
567.2 g/mol | |
Source | PubChem | |
URL | https://pubchem.ncbi.nlm.nih.gov | |
Description | Data deposited in or computed by PubChem | |
CAS No. |
866923-62-8 | |
Record name | 2-(1-((1-(3-((E)-2-(7-Chloroquinolin-2-yl)ethenyl)phenyl)-3-(2-(2-hydroxypropan-2-yl)phenyl)propyl)sulfanylmethyl)cyclopropyl)acetonitrile | |
Source | ChemIDplus | |
URL | https://pubchem.ncbi.nlm.nih.gov/substance/?source=chemidplus&sourceid=0866923628 | |
Description | ChemIDplus is a free, web search system that provides access to the structure and nomenclature authority files used for the identification of chemical substances cited in National Library of Medicine (NLM) databases, including the TOXNET system. | |
Record name | 2-(1-((1-(3-((E)-2-(7-CHLOROQUINOLIN-2-YL)ETHENYL)PHENYL)-3-(2-(2-HYDROXYPROPAN-2-YL)PHENYL)PROPYL)SULFANYLMETHYL)CYCLOPROPYL)ACETONITRILE | |
Source | FDA Global Substance Registration System (GSRS) | |
URL | https://gsrs.ncats.nih.gov/ginas/app/beta/substances/YS7B2ZWL9Z | |
Description | The FDA Global Substance Registration System (GSRS) enables the efficient and accurate exchange of information on what substances are in regulated products. Instead of relying on names, which vary across regulatory domains, countries, and regions, the GSRS knowledge base makes it possible for substances to be defined by standardized, scientific descriptions. | |
Explanation | Unless otherwise noted, the contents of the FDA website (www.fda.gov), both text and graphics, are not copyrighted. They are in the public domain and may be republished, reprinted and otherwise used freely by anyone without the need to obtain permission from FDA. Credit to the U.S. Food and Drug Administration as the source is appreciated but not required. | |
Retrosynthesis Analysis
AI-Powered Synthesis Planning: Our tool employs the Template_relevance Pistachio, Template_relevance Bkms_metabolic, Template_relevance Pistachio_ringbreaker, Template_relevance Reaxys, Template_relevance Reaxys_biocatalysis model, leveraging a vast database of chemical reactions to predict feasible synthetic routes.
One-Step Synthesis Focus: Specifically designed for one-step synthesis, it provides concise and direct routes for your target compounds, streamlining the synthesis process.
Accurate Predictions: Utilizing the extensive PISTACHIO, BKMS_METABOLIC, PISTACHIO_RINGBREAKER, REAXYS, REAXYS_BIOCATALYSIS database, our tool offers high-accuracy predictions, reflecting the latest in chemical research and data.
Strategy Settings
Precursor scoring | Relevance Heuristic |
---|---|
Min. plausibility | 0.01 |
Model | Template_relevance |
Template Set | Pistachio/Bkms_metabolic/Pistachio_ringbreaker/Reaxys/Reaxys_biocatalysis |
Top-N result to add to graph | 6 |
Feasible Synthetic Routes
Disclaimer and Information on In-Vitro Research Products
Please be aware that all articles and product information presented on BenchChem are intended solely for informational purposes. The products available for purchase on BenchChem are specifically designed for in-vitro studies, which are conducted outside of living organisms. In-vitro studies, derived from the Latin term "in glass," involve experiments performed in controlled laboratory settings using cells or tissues. It is important to note that these products are not categorized as medicines or drugs, and they have not received approval from the FDA for the prevention, treatment, or cure of any medical condition, ailment, or disease. We must emphasize that any form of bodily introduction of these products into humans or animals is strictly prohibited by law. It is essential to adhere to these guidelines to ensure compliance with legal and ethical standards in research and experimentation.