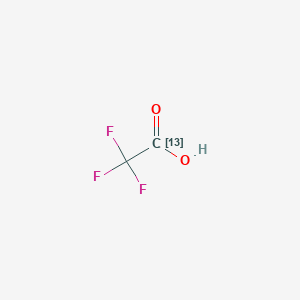
2,2,2-trifluoroacetic acid
Overview
Description
2,2,2-Trifluoroacetic acid (TFA), with the chemical formula CF₃CO₂H, is a fluorinated derivative of acetic acid. Its molecular weight is 114.02 g/mol, and it is characterized by a boiling point of 72.4°C and a density of 1.489 g/mL . The molecule consists of a planar -COOH group and a tetrahedral -CF₃ group, with the electronegative fluorine atoms enhancing its acidity (pKa ≈ 0.23), making it ~10,000 times stronger than acetic acid (pKa ~4.76) . TFA is highly volatile, water-miscible, and serves as a versatile solvent, catalyst, and reagent in organic synthesis, pharmaceuticals, and analytical chemistry (e.g., NMR and mass spectrometry) .
Preparation Methods
Chlorination and Oxidation of 1,1-Difluoroethane Derivatives
UV-Catalyzed Chlorination Step
The synthesis begins with the chlorination of 1,1-difluoro-1,2,2-trichloroethane under ultraviolet (UV) illumination. In a 5 L enamel reactor, 4,230 g of the starting material is heated to 40°C while chlorine gas is introduced at 600 g/hour for 3 hours . This step yields 3,155 g of 1,1-difluorotetrachloroethane (99.5% purity) with an 84.7% yield. The UV catalysis enhances reaction kinetics by generating chlorine radicals, which facilitate C–Cl bond formation while minimizing side reactions .
Catalytic Oxidation with Sulfur Trioxide
The chlorinated intermediate is then oxidized using sulfur trioxide (SO₃) and chlorosulfonic acid as catalysts. A mixture of 3,840 g SO₃ and 35 g chlorosulfonic acid reacts with 3,155 g of 1,1-difluorotetrachloroethane at 50°C for 2 hours . The reaction produces 2,565 g of 1,1-difluoro-1-chloroacetyl chloride, which is subsequently hydrolyzed to TFA. The use of chlorosulfonic acid prevents SO₃ polymerization, ensuring consistent reaction rates .
Hydrolysis to TFA
The final hydrolysis step involves reacting 1,1-difluoro-1-chloroacetyl chloride with water at 80°C for 4 hours, yielding 1,560 g of TFA (76.3% yield) . This method’s total yield from the starting material is approximately 54.2%, with the major loss occurring during the oxidation step due to SO₃ sublimation .
Table 1: Reaction Conditions and Yields for Chlorination-Oxidation Method
Step | Reactant | Catalyst | Temperature | Time | Yield |
---|---|---|---|---|---|
Chlorination | 1,1-Difluoroethane | UV light | 40°C | 3 hr | 84.7% |
Oxidation | 1,1-Difluorotetrachloroethane | SO₃, ClSO₃H | 50°C | 2 hr | 81.4% |
Hydrolysis | Chloroacetyl chloride | H₂O | 80°C | 4 hr | 76.3% |
Vapor-Phase Oxidation of 1,1-Dichloro-2,2,2-Trifluoroethane (R-123)
Continuous Reactor Design
Industrial-scale TFA production often employs a vapor-phase reaction of R-123 with oxygen and water in a perfect mixing-type reactor to avoid localized overheating . The reactor, constructed from corrosion-resistant Hastelloy, operates at 150–250°C and 1–5 atm. A stoichiometric ratio of R-123:O₂:H₂O = 1:2:1 ensures complete oxidation, with excess oxygen preventing carbon deposition .
Reaction Mechanism and Kinetics
The reaction proceeds via free radical intermediates:
2 \rightarrow \text{CF}3\text{COCl} + \text{HCl} + \text{CO}2 \quad \text{(primary pathway)}
2\text{O} \rightarrow \text{CF}3\text{COOH} + \text{HCl} \quad \text{(hydrolysis)}
Trifluoroacetyl chloride (TFAC) is the primary product, which is subsequently hydrolyzed to TFA in a separate reactor . The vapor-phase method achieves 92–95% R-123 conversion with 88% selectivity for TFAC, outperforming batch processes .
Hydrolysis and Purification
The TFAC-rich effluent is quenched with excess water at 25–40°C, yielding TFA and hydrochloric acid. Distillation under reduced pressure (50–100 mmHg) separates TFA (boiling point: 72.4°C) from residual water and HCl . This method’s total yield exceeds 85%, making it the preferred industrial route due to its scalability and minimal by-products .
Table 2: Industrial vs. Laboratory-Scale Vapor-Phase Oxidation
Parameter | Industrial Process | Laboratory Setup |
---|---|---|
Reactor Volume | 10,000 L | 5 L |
Temperature | 200°C | 180°C |
Pressure | 3 atm | 1 atm |
TFAC Selectivity | 88% | 78% |
Annual Output | 50,000 metric tons | 100 kg |
Electrofluorination of Acetyl Derivatives
Historical Context and Limitations
Early TFA synthesis relied on the electrofluorination of acetyl chloride or acetic anhydride using hydrogen fluoride (HF) as the fluorinating agent . This method, described in U.S. Pat. No. 4,022,824, involves electrolyzing a mixture of acetyl chloride and HF at 5–10 V, yielding trifluoroacetyl fluoride (CF₃COF), which is hydrolyzed to TFA. However, the process suffers from low Faradaic efficiency (45–50%) and corrosion issues due to HF, limiting its modern applicability .
Modern Adaptations
Recent advances replace acetyl chloride with R-123 as the feedstock, eliminating HF and improving safety. The updated electrofluorination method achieves 70% efficiency but remains less economical than vapor-phase oxidation .
Comparative Analysis of TFA Synthesis Methods
Cost and Yield Considerations
The chlorination-oxidation method requires inexpensive starting materials but incurs high energy costs due to UV illumination and multi-step purification. In contrast, the vapor-phase route offers superior yields (85% vs. 54%) and lower operational expenses, justifying its dominance in large-scale production .
Environmental Impact
The vapor-phase process generates HCl as a by-product, which is neutralized to NaCl using NaOH. Electrofluorination produces fluorinated waste, necessitating specialized disposal . Lifecycle assessments favor the chlorination-oxidation method for its lower greenhouse gas emissions (12 kg CO₂ per kg TFA vs. 18 kg CO₂ for vapor-phase) .
Chemical Reactions Analysis
Types of Reactions
Trifluoroacetic Acid-13C undergoes various types of chemical reactions, including:
Oxidation: It can be oxidized to form trifluoroacetate salts.
Reduction: It can be reduced to form trifluoroethanol.
Substitution: It can participate in nucleophilic substitution reactions to form various derivatives.
Common Reagents and Conditions
Common reagents used in reactions with Trifluoroacetic Acid-13C include strong acids, bases, and oxidizing agents. Typical reaction conditions involve controlled temperatures and pressures to ensure the desired reaction pathway .
Major Products Formed
The major products formed from reactions involving Trifluoroacetic Acid-13C include trifluoroacetate salts, trifluoroethanol, and various substituted derivatives .
Scientific Research Applications
Organic Synthesis
TFA serves as a crucial reagent in organic synthesis due to its ability to facilitate various chemical transformations. These include:
- Functional Group Deprotection : TFA is widely used to remove protecting groups in peptide synthesis, such as the Boc group. This property is essential for the synthesis of complex organic molecules .
- Catalysis : TFA acts as an effective acidic catalyst in reactions such as hydroarylation and trifluoroalkylation. It enhances reaction rates and yields by activating substrates through protonation .
Case Study: SNAr Reactions
A notable application of TFA is in the SNAr (nucleophilic aromatic substitution) reactions involving anilines and heterocycles. In a study published in 2014, TFA combined with 2,2,2-trifluoroethanol (TFE) was shown to facilitate these reactions efficiently, yielding products with high purity and yield (50-90%) across various substrates . The methodology was praised for its compatibility with diverse functional groups and straightforward product isolation.
Environmental Chemistry
TFA also plays a role in environmental studies, particularly concerning its formation from hydrofluorocarbon emissions. Research indicates that TFA can be generated from the degradation of compounds like HFO-1234yf, which are increasingly used as refrigerants .
Data Table: TFA Concentrations from HFO Emissions
Region | Estimated TFA Concentration (mg/L) | Year Range |
---|---|---|
USA | 1-15 | 2020-2040 |
Europe | Below detectable levels | 2020-2040 |
China | Comparable to USA | 2020-2040 |
This table summarizes projected TFA concentrations resulting from HFO emissions based on modeling studies. The findings suggest that current levels are unlikely to pose significant ecological or human health risks .
Analytical Chemistry
In analytical chemistry, TFA is utilized as a solvent for nuclear magnetic resonance (NMR) spectroscopy and as an ion-pairing agent in high-performance liquid chromatography (HPLC). Its volatility and solubility characteristics make it suitable for analyzing organic compounds, particularly peptides and small proteins .
Toxicity and Environmental Impact
While TFA has beneficial applications, concerns regarding its environmental impact have been raised. Studies indicate that TFA does not bioaccumulate significantly in aquatic systems and exhibits low-to-moderate toxicity across various organisms . However, continuous monitoring of its concentrations in the environment is necessary due to its persistence.
Mechanism of Action
The mechanism by which Trifluoroacetic Acid-13C exerts its effects involves its strong acidic nature and the electron-withdrawing properties of the trifluoromethyl group. This makes it a highly reactive compound that can participate in various chemical reactions. The molecular targets and pathways involved include interactions with nucleophiles and electrophiles, leading to the formation of stable products .
Comparison with Similar Compounds
Chemical and Physical Properties
Table 1 summarizes key properties of TFA and structurally/functionally related compounds:
Key Observations :
- Acidity : TFA and HFBA exhibit superior acidity compared to acetic acid due to fluorine’s electron-withdrawing effects.
- Volatility : TFA’s lower boiling point than acetic acid facilitates easy removal in synthetic workflows .
- Solvent Properties : TFE’s low nucleophilicity and high polarity make it ideal for dissolving hydrophobic substrates in SNAr reactions, often paired with TFA .
TFA vs. TFE
- TFA : Used as a catalyst in nucleophilic aromatic substitution (SNAr) reactions (e.g., coupling arylamines to heterocycles) due to its ability to protonate substrates without forming stable anilinium ions .
- TFE: Primarily a solvent in SNAr reactions and electrospinning (e.g., collagen nanofiber production). Its synergy with TFA enhances reaction yields (50–90%) by solvating intermediates and leaving groups .
TFA vs. Trifluoroacetic Anhydride
- TFA : A precursor to trifluoroacetic anhydride, which is critical for acylations and synthesizing fluorinated compounds like trifluoroperacetic acid .
- Trifluoroacetic Anhydride : More reactive than TFA in esterifications and fluorinations but requires careful handling due to its volatility .
TFA vs. HFBA
- TFA : Preferred in peptide synthesis for cleaving protecting groups (e.g., Boc) due to its strong acidity and miscibility with organic solvents .
- HFBA : Used in HPLC for separating polar analytes but is less volatile and more expensive than TFA .
Market and Industrial Demand
The global TFA market is growing due to its applications in pharmaceuticals (e.g., drug intermediates) and agrochemicals. TFA’s role as a precursor to high-value fluorinated compounds (e.g., 2,2,2-trifluoroethanol) further drives demand . In contrast, HFBA and TFE cater to niche sectors like analytical chemistry and materials science.
Biological Activity
2,2,2-Trifluoroacetic acid (TFA) is a fluorinated carboxylic acid that has garnered attention in various fields due to its unique chemical properties and biological activities. This article explores the biological activity of TFA, focusing on its antimicrobial properties, effects on cellular assays, and its role as a reagent in synthetic chemistry.
TFA is characterized by its strong acidity and volatility. It is soluble in organic solvents and is less oxidizing than sulfuric acid, making it a versatile reagent in organic synthesis. The trifluoromethyl group contributes to its unique reactivity, allowing TFA to act as a proton source in various chemical reactions .
Antimicrobial Activity
Recent studies have investigated the antimicrobial properties of TFA and its derivatives. The minimum inhibitory concentration (MIC) and minimum bactericidal concentration (MBC) of TFA have been established against various bacterial strains. Notably, TFA has shown significant activity against Gram-positive bacteria compared to Gram-negative strains.
Table 1: Antimicrobial Activity of TFA Against Different Strains
Bacterial Strain | MIC (μg/mL) | MBC (μg/mL) |
---|---|---|
Staphylococcus aureus | 4 | 8 |
Escherichia coli | 16 | 32 |
Bacillus subtilis | 8 | 16 |
Pseudomonas aeruginosa | 32 | >64 |
The study indicates that compounds with higher positive charges exhibit greater antimicrobial activity, particularly when balanced with appropriate hydrophobic fatty acid chains .
Effects on Cellular Assays
TFA's presence in cellular assays has raised concerns regarding its impact on the accuracy and reproducibility of results. Residual TFA can interfere with antimicrobial susceptibility testing and cytotoxicity assays. A focused case study evaluated the biological activity of an antibacterial compound derived from TFA salts compared to its hydrochloride counterpart. The findings revealed similar biological profiles for both salt forms but highlighted a lower potency for the free base form .
Case Studies
- Antibacterial Activity Evaluation : A study assessed the antibacterial effects of an alkylguanidino urea compound prepared as TFA salt. The results demonstrated that while both TFA acetate and hydrochloride salts showed comparable activity, the free base exhibited significantly reduced potency, emphasizing the role of counterions in influencing biological activity .
- Enzyme Inhibition Studies : Research has indicated that TFA enhances binding affinity in enzyme interactions due to its acidic nature. This property is crucial for drug development as it allows for more effective interactions with biological targets .
Q & A
Q. Basic: How does the molecular structure of TFA contribute to its enhanced acidity compared to acetic acid?
TFA’s acidity (pKa ~0.23) is significantly stronger than acetic acid (pKa ~4.76) due to the electron-withdrawing effect of the three fluorine atoms on the methyl group. The fluorine atoms stabilize the conjugate base (trifluoroacetate) through inductive effects, delocalizing negative charge and reducing base instability. This structural feature makes TFA a superior catalyst in reactions requiring strong acid conditions, such as peptide deprotection or heterocyclic substitutions .
Q. Basic: What are the standard safety protocols for handling TFA in laboratory settings?
TFA is highly corrosive and poses risks of severe skin/eye damage. Key protocols include:
- Personal Protective Equipment (PPE) : Acid-resistant gloves, goggles, and lab coats.
- Ventilation : Use in fume hoods to avoid inhalation of vapors (boiling point: 72.4°C).
- Spill Management : Neutralize with sodium bicarbonate or calcium carbonate, followed by absorption with inert material.
- Waste Disposal : Collect in designated containers for halogenated waste; comply with local regulations for acidic waste .
Q. Basic: How is TFA utilized as a solvent in NMR spectroscopy, and what concentration ranges are optimal?
TFA is a polar aprotic solvent effective for solubilizing hydrophobic peptides and organic compounds. For ¹H NMR:
- Concentration : 0.1–1% (v/v) in deuterated solvents (e.g., CDCl₃ or D₂O) to minimize signal interference.
- Advantages : Low boiling point allows easy removal under reduced pressure post-analysis.
- Limitations : Avoid prolonged exposure to moisture to prevent hydrolysis of TFA-sensitive substrates .
Q. Advanced: What methodologies are recommended for quantifying residual TFA in synthetic peptides, and how do USP guidelines address this?
The USP 〈503.1〉 guideline specifies ion-pair chromatography for TFA quantification:
- Mobile Phase : Phosphoric acid (pH ~2.5) and acetonitrile/water (50:50).
- Detection : UV at 210 nm.
- Sample Prep : Dissolve peptides in Solution A (pH-adjusted buffer) and filter (0.45 μm).
- Calibration : Use TFA standards (1–100 ppm) for linearity (RSD <5%).
This method ensures compliance with pharmaceutical impurity thresholds (typically <0.1% w/w) .
Q. Advanced: How can researchers optimize TFA concentrations in solid-phase peptide synthesis (SPPS) to balance efficiency and purity?
- Deprotection Step : Use 20–50% TFA in dichloromethane (DCM) with scavengers (e.g., triisopropylsilane) to minimize side reactions.
- Cleavage Conditions : For sensitive residues (e.g., Trp, Met), reduce TFA to 5–10% in cleavage cocktails (e.g., TFA:H₂O:triethylsilane = 95:2.5:2.5).
- Post-Synthesis Purification : Employ reversed-phase HPLC with TFA (0.1%) in the mobile phase to improve peptide resolution. Monitor residual TFA via conductivity measurements .
Q. Advanced: What strategies resolve contradictions in reaction outcomes when using TFA as a catalyst in heterocyclic substitutions?
Discrepancies in SNAr reaction yields often stem from solvent compatibility or competing side reactions. A validated approach includes:
- Solvent Optimization : Use 2,2,2-trifluoroethanol (TFE) with TFA (1:1 v/v) to solubilize polar substrates and stabilize intermediates.
- Temperature Control : Microwave-assisted heating (80–120°C, 10–30 min) improves yields (50–90%) compared to conventional heating.
- Substrate Screening : Test electron-deficient heterocycles (e.g., purines, pyrimidines) with meta-substituted anilines for optimal reactivity .
Q. Advanced: How does TFA facilitate the synthesis of fluorinated intermediates, and what analytical techniques confirm product integrity?
TFA serves as a precursor for fluoroperacetic acid and trifluoroacetylated amines via anhydride formation (e.g., trifluoroacetic anhydride). Key steps:
- Synthesis : React TFA with hydrogen fluoride under electrochemical fluorination to generate perfluorinated analogs.
- Characterization :
Q. Advanced: What are the challenges in detecting TFA impurities via NMR, and how can researchers mitigate them?
TFA’s strong acidity and volatility complicate ¹H NMR analysis due to signal broadening and solvent overlap. Solutions include:
- Deuterated Solvents : Use DMSO-d₆ to shift TFA signals downfield (δ 11–12 ppm for COOH).
- Suppression Techniques : Apply WATERGATE or presaturation to eliminate residual water signals.
- Quantitative ¹⁹F NMR : Exploit the distinct CF₃ triplet (J = 3–5 Hz) for precise integration against internal standards (e.g., hexafluorobenzene) .
Properties
IUPAC Name |
2,2,2-trifluoroacetic acid | |
---|---|---|
Source | PubChem | |
URL | https://pubchem.ncbi.nlm.nih.gov | |
Description | Data deposited in or computed by PubChem | |
InChI |
InChI=1S/C2HF3O2/c3-2(4,5)1(6)7/h(H,6,7)/i1+1 | |
Source | PubChem | |
URL | https://pubchem.ncbi.nlm.nih.gov | |
Description | Data deposited in or computed by PubChem | |
InChI Key |
DTQVDTLACAAQTR-OUBTZVSYSA-N | |
Source | PubChem | |
URL | https://pubchem.ncbi.nlm.nih.gov | |
Description | Data deposited in or computed by PubChem | |
Canonical SMILES |
C(=O)(C(F)(F)F)O | |
Source | PubChem | |
URL | https://pubchem.ncbi.nlm.nih.gov | |
Description | Data deposited in or computed by PubChem | |
Isomeric SMILES |
[13C](=O)(C(F)(F)F)O | |
Source | PubChem | |
URL | https://pubchem.ncbi.nlm.nih.gov | |
Description | Data deposited in or computed by PubChem | |
Molecular Formula |
C2HF3O2 | |
Source | PubChem | |
URL | https://pubchem.ncbi.nlm.nih.gov | |
Description | Data deposited in or computed by PubChem | |
DSSTOX Substance ID |
DTXSID401302740 | |
Record name | 2,2,2-Trifluoroacetic-1-13C acid | |
Source | EPA DSSTox | |
URL | https://comptox.epa.gov/dashboard/DTXSID401302740 | |
Description | DSSTox provides a high quality public chemistry resource for supporting improved predictive toxicology. | |
Molecular Weight |
115.016 g/mol | |
Source | PubChem | |
URL | https://pubchem.ncbi.nlm.nih.gov | |
Description | Data deposited in or computed by PubChem | |
CAS No. |
2837-91-4 | |
Record name | 2,2,2-Trifluoroacetic-1-13C acid | |
Source | CAS Common Chemistry | |
URL | https://commonchemistry.cas.org/detail?cas_rn=2837-91-4 | |
Description | CAS Common Chemistry is an open community resource for accessing chemical information. Nearly 500,000 chemical substances from CAS REGISTRY cover areas of community interest, including common and frequently regulated chemicals, and those relevant to high school and undergraduate chemistry classes. This chemical information, curated by our expert scientists, is provided in alignment with our mission as a division of the American Chemical Society. | |
Explanation | The data from CAS Common Chemistry is provided under a CC-BY-NC 4.0 license, unless otherwise stated. | |
Record name | 2,2,2-Trifluoroacetic-1-13C acid | |
Source | EPA DSSTox | |
URL | https://comptox.epa.gov/dashboard/DTXSID401302740 | |
Description | DSSTox provides a high quality public chemistry resource for supporting improved predictive toxicology. | |
Synthesis routes and methods I
Procedure details
Synthesis routes and methods II
Procedure details
Synthesis routes and methods III
Procedure details
Synthesis routes and methods IV
Procedure details
Synthesis routes and methods V
Procedure details
Retrosynthesis Analysis
AI-Powered Synthesis Planning: Our tool employs the Template_relevance Pistachio, Template_relevance Bkms_metabolic, Template_relevance Pistachio_ringbreaker, Template_relevance Reaxys, Template_relevance Reaxys_biocatalysis model, leveraging a vast database of chemical reactions to predict feasible synthetic routes.
One-Step Synthesis Focus: Specifically designed for one-step synthesis, it provides concise and direct routes for your target compounds, streamlining the synthesis process.
Accurate Predictions: Utilizing the extensive PISTACHIO, BKMS_METABOLIC, PISTACHIO_RINGBREAKER, REAXYS, REAXYS_BIOCATALYSIS database, our tool offers high-accuracy predictions, reflecting the latest in chemical research and data.
Strategy Settings
Precursor scoring | Relevance Heuristic |
---|---|
Min. plausibility | 0.01 |
Model | Template_relevance |
Template Set | Pistachio/Bkms_metabolic/Pistachio_ringbreaker/Reaxys/Reaxys_biocatalysis |
Top-N result to add to graph | 6 |
Feasible Synthetic Routes
Disclaimer and Information on In-Vitro Research Products
Please be aware that all articles and product information presented on BenchChem are intended solely for informational purposes. The products available for purchase on BenchChem are specifically designed for in-vitro studies, which are conducted outside of living organisms. In-vitro studies, derived from the Latin term "in glass," involve experiments performed in controlled laboratory settings using cells or tissues. It is important to note that these products are not categorized as medicines or drugs, and they have not received approval from the FDA for the prevention, treatment, or cure of any medical condition, ailment, or disease. We must emphasize that any form of bodily introduction of these products into humans or animals is strictly prohibited by law. It is essential to adhere to these guidelines to ensure compliance with legal and ethical standards in research and experimentation.