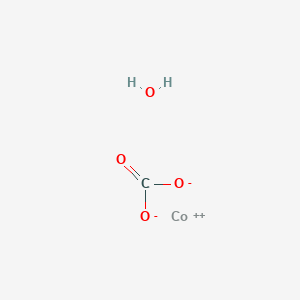
Cobalt(II) carbonate hydrate
Overview
Description
Cobalt(II) carbonate hydrate (CoCO₃·xH₂O), often abbreviated as CCHH, is a hydrated form of cobalt carbonate with a variable water content depending on synthesis conditions. It crystallizes in a rhombohedral structure (JCPDS No. 48-0084) and exhibits a pink-to-violet coloration . The compound is typically synthesized via hydrothermal methods using cobalt chloride hexahydrate and urea as precursors, producing a three-dimensional (3D) interconnected microstructure with high specific surface area (~100 m²/g) . This morphology makes it suitable for gas-sensing applications, though its intrinsic low conductivity limits performance unless hybridized with conductive materials like reduced graphene oxide (RGO) .
CCHH is also a precursor for Co₃O₄ synthesis through thermal decomposition at 300–500°C .
Preparation Methods
Cobalt(II) carbonate hydrate can be synthesized by combining solutions of cobalt(II) sulfate and sodium bicarbonate. The reaction proceeds as follows:
CoSO4+2NaHCO3→CoCO3+Na2SO4+H2O+CO2
This reaction is commonly used in the precipitation of cobalt from an extract of its roasted ores .
Chemical Reactions Analysis
Reactions with Acids
Cobalt(II) carbonate hydrate reacts vigorously with mineral acids, producing cobalt(II) salts, water, and carbon dioxide.
Example: Reaction with Hydrochloric Acid
Key Observations:
- Reaction kinetics depend on particle size and hydration state .
- Acid strength influences CO₂ evolution rate .
Thermal Decomposition
Decomposition pathways vary with temperature and atmosphere:
Kinetic Analysis:
- Non-isothermal studies using the Coats-Redfern method reveal multi-stage decomposition .
- Deconvolution of overlapping steps (e.g., dehydroxylation and decarbonation) requires Fraser–Suzuki fitting .
Oxidation Reactions
This compound serves as a precursor for mixed-valence cobalt oxides:
Coordination Chemistry
Reacts with organic ligands to form complexes:
- Acetylacetonate Complex :
(acacH = acetylacetone) . - Dipicolinate Synthesis :
Reacts with dipicolinic acid and 4,4′-bipyridine to yield transition metal dipicolinates .
Comparative Reactivity
Property | This compound | Nickel(II) Carbonate | Copper(II) Carbonate |
---|---|---|---|
Acid Reactivity | Rapid CO₂ evolution | Moderate | Slow (malachite form) |
Decomposition Temp (°C) | 136–270 | 200–300 | 290–350 |
Oxide Product | Co₃O₄, CoO | NiO | CuO |
Scientific Research Applications
Scientific Research Applications
Cobalt(II) carbonate hydrate has several notable applications in scientific research, including:
Catalysis
This compound is utilized as a precursor for various cobalt-based catalysts. These catalysts are essential in industrial processes such as Fischer-Tropsch synthesis, which converts carbon monoxide and hydrogen into liquid hydrocarbons. The compound can also be used to synthesize cobalt carbonyl and cobalt salts, which are critical for numerous catalytic reactions .
Material Science
In material science, this compound is employed in the synthesis of transition metal dipicolinates by reacting with dipicolinic acid and 4,4′-bipyridine. These compounds are significant for their magnetic properties and potential applications in data storage technologies .
Battery Manufacturing
Cobalt compounds, including this compound, are integral to the production of lithium-ion batteries due to their excellent electrochemical properties. They contribute to the performance of cathode materials, enhancing energy density and cycle stability .
Pigment Production
The compound is also used as a pigment in ceramics and glass manufacturing. Its vibrant color makes it suitable for use in decorative applications, including camouflage coatings .
Agricultural Applications
This compound serves as a microelement fertilizer in agriculture, providing essential nutrients to crops. Its role as a feed additive helps improve livestock health by supplying necessary trace elements .
Case Study 1: Catalytic Applications
Research has demonstrated that cobalt-based catalysts derived from this compound exhibit high activity in the hydrogenation of carbon dioxide to methanol. The catalytic performance was attributed to the specific surface area and active sites present in the synthesized materials.
Case Study 2: Battery Performance
A study evaluated the impact of using this compound in lithium-ion batteries, revealing that cells incorporating this compound showed improved charge-discharge cycles compared to those using traditional cobalt oxide materials. This enhancement is linked to better structural stability during cycling.
Mechanism of Action
The mechanism by which cobalt(II) carbonate hydrate exerts its effects involves its ability to act as a precursor to various cobalt compounds. These compounds can participate in redox reactions, coordination chemistry, and catalysis. For instance, cobalt(II) carbonate can be converted to cobalt(II) oxide, which is a key component in many catalytic processes .
Comparison with Similar Compounds
Cobalt(II) Carbonate (Anhydrous, CoCO₃)
The hydrated form’s 3D structure enhances gas adsorption compared to the denser anhydrous form, but both suffer from low conductivity. Anhydrous CoCO₃ is more thermally stable, decomposing at ~350°C versus ~200°C for CCHH .
Nickel-Cobalt Carbonate Hydroxide Hydrate (Ni-Co CHH)
Bimetallic Ni-Co CHH (e.g., NiₓCo₁₋ₓ(CO₃)₀.₅(OH)·0.11H₂O) shows superior electrochemical performance for supercapacitors compared to CCHH. Key differences include:
- Electronic Properties : Ni²⁺ exhibits mixed spin states, while Co²⁺ remains in low-spin configurations. Oxygen vacancies preferentially form around Ni sites, enhancing charge storage .
- Capacitance: Ni-Co CHH achieves ~1,200 F/g at 1 A/g, outperforming monometallic CCHH (~400 F/g) due to synergistic redox activity .
- Structural Stability : Higher Ni content introduces octahedral distortion, reducing cycle life but increasing surface reactivity .
Manganese(II) Carbonate Hydrate (MnCO₃·xH₂O)
MnCO₃·xH₂O’s antiferromagnetic properties and lower toxicity make it preferable for lithium-ion battery anodes, while CCHH’s redox activity favors catalytic applications.
Nickel(II) Carbonate Basic Hydrate (NiCO₃·2Ni(OH)₂·xH₂O)
This compound shares a layered hydroxide-carbonate structure but differs in:
- Thermal Stability : Decomposes at ~280°C, releasing CO₂ and H₂O, versus CCHH’s ~200°C .
- Hazards: Classified as a carcinogen (Ni content) with higher toxicity than CCHH .
- Use Cases : Primarily in electroplating and catalysts, unlike CCHH’s gas-sensing niche .
Key Research Findings
- Gas Sensing : CCHH-RGO hybrids achieve a 9% response to 1 ppm NH₃ at 0.4 wt% RGO, outperforming pure CCHH (1% response) .
- Supercapacitors : Ni-Co CHH delivers 92% capacitance retention after 5,000 cycles, leveraging oxygen vacancies for stability .
- Thermal Behavior : CCHH decomposes to Co₃O₄ at lower temperatures (~300°C) than anhydrous CoCO₃ (~350°C), enabling energy-efficient catalyst synthesis .
Biological Activity
Cobalt(II) carbonate hydrate (CoCO₃·xH₂O) is an inorganic compound that plays a significant role in various biological and biochemical processes. This article explores its biological activity, mechanisms of action, safety considerations, and applications in scientific research.
Overview of this compound
This compound is a pink paramagnetic solid primarily used as a reagent in the synthesis of transition metal dipicolinates and other cobalt-based compounds. It is also utilized in various applications, including catalysis and battery manufacturing due to its electrochemical properties .
Biological Significance
Cobalt is an essential trace element for many organisms, primarily due to its role as a component of vitamin B12 (cobalamin). The biological activity of cobalt compounds, including this compound, is closely linked to their ability to participate in enzymatic reactions and influence metabolic pathways.
- Absorption and Distribution : Cobalt from dietary sources is absorbed in the gastrointestinal tract. Once absorbed, cobalt can be deposited in various tissues, particularly the liver and kidneys, where it may exert biological effects .
- Role in Cobalamin Synthesis : In ruminants, cobalt(II) carbonate hydroxide (a related compound) facilitates the synthesis of cobalamin by the gastrointestinal microbiota. This process is crucial for maintaining normal metabolic functions .
Case Studies and Research Findings
Safety and Hazards
This compound poses several health risks:
- Irritation : It is known to cause skin and eye irritation upon contact.
- Respiratory Sensitization : Inhalation of cobalt dust can lead to respiratory sensitization and other pulmonary issues.
- Carcinogenic Potential : Prolonged exposure to cobalt compounds has been linked to an increased risk of lung cancer .
Applications in Research
This compound serves as a precursor for various chemical syntheses:
- Catalyst Preparation : It is used to prepare cobalt-based catalysts essential for industrial processes.
- Material Science : The compound is involved in the synthesis of transition metal dipicolinates, which have applications in coordination chemistry .
Comparative Data Table
Property | Value/Description |
---|---|
Molecular Formula | CoCO₃·xH₂O |
Appearance | Pink paramagnetic solid |
Solubility | Insoluble in water; soluble in mineral acids |
Cobalt Content | ≥45.5% (by titration complexometric method) |
Toxicity | Harmful if swallowed; may cause allergic reactions |
Q & A
Basic Research Questions
Q. What are the recommended synthesis methods for Cobalt(II) carbonate hydrate, and how do reaction conditions influence purity and morphology?
this compound is typically synthesized via wet chemical routes using aqueous solutions of cobalt salts (e.g., Co(NO₃)₂ or CoCl₂) and carbonate precursors (e.g., Na₂CO₃). Reaction parameters such as pH, temperature, and stirring rate critically affect crystallinity and particle size. For example, slow precipitation at neutral pH (~7) and moderate temperatures (50–80°C) yields well-defined nanostructures, while rapid mixing may produce amorphous phases. Post-synthesis washing with deionized water and ethanol is essential to remove ionic byproducts .
Q. How can researchers characterize the hydration state and thermal stability of this compound?
Thermogravimetric analysis (TGA) coupled with differential scanning calorimetry (DSC) is used to determine hydration levels and decomposition pathways. For instance, heating from 25°C to 600°C under inert atmosphere reveals stepwise mass loss corresponding to water release (25–150°C) and carbonate decomposition to Co₃O₄ (>250°C). FTIR spectroscopy (400–4000 cm⁻¹) identifies O-H stretching (~3400 cm⁻¹) and carbonate vibrational modes (~1400 cm⁻¹) to confirm hydration and structural integrity .
Q. What analytical techniques are suitable for quantifying cobalt and carbonate content in the compound?
Gravimetric analysis via thermal decomposition (e.g., heating to form Co₃O₄) provides cobalt content, while redox titration with KMnO₄ under acidic conditions quantifies oxalate or carbonate ions. For precise elemental ratios, inductively coupled plasma mass spectrometry (ICP-MS) and combustion analysis (CHNS/O) are recommended. Cross-validation with X-ray diffraction (XRD) ensures phase purity .
Advanced Research Questions
Q. How do variations in synthesis parameters affect the electronic structure and spin states of cobalt in this compound?
X-ray absorption near-edge structure (XANES) at Co K- and L-edges reveals oxidation states and ligand field effects. Studies show Co²⁺ in this compound adopts a low-spin configuration (t₂g⁶e_g¹) due to strong octahedral crystal field splitting. Synchrotron-based spectroscopy further identifies ligand-field splitting energies (~1.5–2.0 eV), comparable to CoO, which influence redox activity and catalytic properties .
Q. What methodologies resolve contradictions in reported crystal structures of this compound?
Discrepancies arise from differences in hydration levels and cation substitution (e.g., Ni²⁺ doping). Synchrotron XRD with Rietveld refinement is critical for accurate structural determination. For example, refining occupancy factors and lattice parameters confirms a monoclinic structure (space group C2/m) for the hydrated phase, while anhydrous forms may adopt trigonal symmetry. Pair distribution function (PDF) analysis can further resolve local structural disorder .
Q. How do oxygen vacancies and defects in this compound influence its electrochemical performance?
Oxygen vacancies, quantified via electron paramagnetic resonance (EPR) or positron annihilation spectroscopy, enhance ionic conductivity and active sites for catalysis. In supercapacitor applications, vacancy-rich nanostructures exhibit higher specific capacitance (e.g., 1120 F/g at 1 A/g) due to improved charge transfer. Controlled calcination under reducing atmospheres (e.g., H₂/Ar) introduces defects while preserving structural integrity .
Q. What strategies mitigate challenges in replicating literature data on this compound’s magnetic properties?
Magnetic susceptibility measurements often conflict due to impurities or incomplete dehydration. Purification via Soxhlet extraction and strict oxygen-free handling (glovebox) are essential. SQUID magnetometry reveals paramagnetic behavior (χ ~ 0.01 emu/g·Oe) at 300 K, transitioning to antiferromagnetic ordering below 15 K. Consistency in sample preparation and measurement protocols (e.g., field-cooled vs. zero-field-cooled) minimizes discrepancies .
Q. Methodological Notes
- Safety : Use PPE (gloves, lab coat) and handle in fume hoods due to potential cobalt toxicity (IARC Group 2B carcinogen) .
- Data Validation : Cross-reference XRD patterns with ICDD databases (e.g., PDF 00-048-0083 for CoCO₃·H₂O) and report hydration levels via TGA mass loss .
Properties
IUPAC Name |
cobalt(2+);carbonate;hydrate | |
---|---|---|
Source | PubChem | |
URL | https://pubchem.ncbi.nlm.nih.gov | |
Description | Data deposited in or computed by PubChem | |
InChI |
InChI=1S/CH2O3.Co.H2O/c2-1(3)4;;/h(H2,2,3,4);;1H2/q;+2;/p-2 | |
Source | PubChem | |
URL | https://pubchem.ncbi.nlm.nih.gov | |
Description | Data deposited in or computed by PubChem | |
InChI Key |
JLAIPADPFFTYLP-UHFFFAOYSA-L | |
Source | PubChem | |
URL | https://pubchem.ncbi.nlm.nih.gov | |
Description | Data deposited in or computed by PubChem | |
Canonical SMILES |
C(=O)([O-])[O-].O.[Co+2] | |
Source | PubChem | |
URL | https://pubchem.ncbi.nlm.nih.gov | |
Description | Data deposited in or computed by PubChem | |
Molecular Formula |
CH2CoO4 | |
Source | PubChem | |
URL | https://pubchem.ncbi.nlm.nih.gov | |
Description | Data deposited in or computed by PubChem | |
DSSTOX Substance ID |
DTXSID70929810 | |
Record name | Cobalt(2+) carbonate--water (1/1/1) | |
Source | EPA DSSTox | |
URL | https://comptox.epa.gov/dashboard/DTXSID70929810 | |
Description | DSSTox provides a high quality public chemistry resource for supporting improved predictive toxicology. | |
Molecular Weight |
136.96 g/mol | |
Source | PubChem | |
URL | https://pubchem.ncbi.nlm.nih.gov | |
Description | Data deposited in or computed by PubChem | |
CAS No. |
137506-60-6 | |
Record name | Cobalt(2+) carbonate--water (1/1/1) | |
Source | EPA DSSTox | |
URL | https://comptox.epa.gov/dashboard/DTXSID70929810 | |
Description | DSSTox provides a high quality public chemistry resource for supporting improved predictive toxicology. | |
Retrosynthesis Analysis
AI-Powered Synthesis Planning: Our tool employs the Template_relevance Pistachio, Template_relevance Bkms_metabolic, Template_relevance Pistachio_ringbreaker, Template_relevance Reaxys, Template_relevance Reaxys_biocatalysis model, leveraging a vast database of chemical reactions to predict feasible synthetic routes.
One-Step Synthesis Focus: Specifically designed for one-step synthesis, it provides concise and direct routes for your target compounds, streamlining the synthesis process.
Accurate Predictions: Utilizing the extensive PISTACHIO, BKMS_METABOLIC, PISTACHIO_RINGBREAKER, REAXYS, REAXYS_BIOCATALYSIS database, our tool offers high-accuracy predictions, reflecting the latest in chemical research and data.
Strategy Settings
Precursor scoring | Relevance Heuristic |
---|---|
Min. plausibility | 0.01 |
Model | Template_relevance |
Template Set | Pistachio/Bkms_metabolic/Pistachio_ringbreaker/Reaxys/Reaxys_biocatalysis |
Top-N result to add to graph | 6 |
Feasible Synthetic Routes
Disclaimer and Information on In-Vitro Research Products
Please be aware that all articles and product information presented on BenchChem are intended solely for informational purposes. The products available for purchase on BenchChem are specifically designed for in-vitro studies, which are conducted outside of living organisms. In-vitro studies, derived from the Latin term "in glass," involve experiments performed in controlled laboratory settings using cells or tissues. It is important to note that these products are not categorized as medicines or drugs, and they have not received approval from the FDA for the prevention, treatment, or cure of any medical condition, ailment, or disease. We must emphasize that any form of bodily introduction of these products into humans or animals is strictly prohibited by law. It is essential to adhere to these guidelines to ensure compliance with legal and ethical standards in research and experimentation.