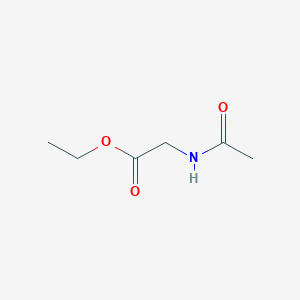
Ethyl acetamidoacetate
Overview
Description
Ethyl acetamidoacetate (CAS: 1906-82-7; molecular formula: C₆H₁₁NO₃) is a bifunctional compound containing both an ester (-COOEt) and an acetamido (-NHCOCH₃) group. It is widely recognized as a peptide mimetic due to its structural similarity to amino acid derivatives, making it valuable in conformational studies using microwave spectroscopy . The compound serves as a critical intermediate in organic synthesis, particularly in the preparation of pharmaceuticals such as indole-2-carboxylic esters (yields: 68–91%) and N-acetyl phenylalanine derivatives (enantiomeric excess >99%) . It also plays a role in synthesizing anti-inflammatory agents like Amtolmetin guacil . Its vaporization enthalpy is reported as 69.4 kJ/mol at ~398 K .
Preparation Methods
Synthetic Routes and Reaction Conditions: Ethyl acetamidoacetate is typically synthesized through the esterification of acetylglycine. The process involves the reaction of acetylglycine with ethanol in the presence of a strongly acidic styrene cation exchange resin. The reaction mixture is refluxed for about three hours, followed by cooling to room temperature. The resulting product is then filtered, and the ethanol is recovered through distillation .
Industrial Production Methods: In industrial settings, the production of this compound follows a similar process but on a larger scale. The use of continuous reactors and efficient recovery systems for ethanol and other solvents ensures high yield and purity of the final product .
Chemical Reactions Analysis
Keto-Enol Tautomerism
Ethyl acetoacetate exhibits keto-enol tautomerism due to its β-keto ester structure:
- Keto form : CH₃C(O)CH₂CO₂C₂H₅
- Enol form : CH₃C(OH)=CHCO₂C₂H₅
Key Data :
Property | Value | Source |
---|---|---|
Enol content (neat, 33°C) | 15% | |
pKa (α-hydrogens) | ~10–11 |
The enol form is stabilized by intramolecular hydrogen bonding and resonance, enabling diverse reactivity .
Reaction Mechanism:
- Deprotonation : Strong bases (e.g., NaH, NaOEt) abstract the α-hydrogen to form an enolate .
- Alkylation : The enolate reacts with alkyl halides (R-X) via SN2 to form alkylated products .
- Hydrolysis/Decarboxylation : Acid or base hydrolysis yields β-keto acids, which decarboxylate to ketones .
Example :
Step | Reagents/Conditions | Product | Yield |
---|---|---|---|
Alkylation with CH₃I | NaOEt, EtOH | CH₃C(O)CH(CH₃)CO₂C₂H₅ | 85% |
Hydrolysis (HCl, Δ) | H₃O⁺, heat | CH₃C(O)CH(CH₃)₂ (3-pentanone) | 75% |
Reduction Reactions
Ethyl acetoacetate reduces to ethyl 3-hydroxybutyrate (β-hydroxy ester):
Reducing Agent | Conditions | Product | Enantioselectivity |
---|---|---|---|
NaBH₄ | EtOH, 0°C → RT | Racemic mixture | None |
Baker’s yeast | Sucrose, 25°C, 24h | Ethyl (S)-3-hydroxybutyrate | >90% ee |
NaBH₄ + L-tartaric acid | THF, 0°C | Ethyl (R)-3-hydroxybutyrate | 85% ee |
Knoevenagel Condensation
Reacts with aldehydes/ketones to form α,β-unsaturated ketones:
Example :
EAA + benzaldehyde → C₆H₅CH=C(CO₂Et)₂ (85% yield, ).
Oxidation Reactions
Chromic acid (H₂CrO₄) oxidizes EAA in acetic acid/water:
Parameter | Value | Source |
---|---|---|
Rate law | First-order in [EAA], [Cr(VI)] | |
Activation energy (ΔH‡) | 8.9 kcal/mol | |
Entropy (ΔS‡) | -41.0 e.u. |
Mechanism : One-electron transfer via Cr(VI) → Cr(III), forming acetic acid and CO₂ .
Scientific Research Applications
Chemical Synthesis
1.1. Indole Derivatives Synthesis
EAA is employed as a key reagent in the synthesis of indole derivatives, which are crucial in pharmaceuticals due to their biological activities. A notable study demonstrated the use of copper nanoparticles immobilized on montmorillonite (Cu-Q-MMT) as a catalyst for synthesizing indole-2-carboxylic esters from ortho-bromobenzaldehydes and EAA. The reaction yielded significant results, achieving up to 85% yield for various substrates, including challenging chlorobenzaldehydes .
Substrate | Yield (%) |
---|---|
2-Chlorobenzaldehyde | 68 |
2,4-Dichlorobenzaldehyde | 75 |
The high catalytic efficiency and stability of Cu-Q-MMT were attributed to the synergistic effects of biquaternary ammonium salts and the nanospace structure of montmorillonite, allowing for effective recycling of the catalyst without significant loss of activity .
1.2. Protein Modification
EAA serves as a reagent for protein modification, aiding in enzyme activity studies and the development of bioconjugates. Its ability to introduce acetamido groups makes it valuable for enhancing solubility and stability in peptide synthesis.
Biological Applications
2.1. Antimicrobial Activity
Research has shown that derivatives synthesized from EAA exhibit promising antimicrobial properties. For instance, indole-2-carboxylic esters derived from EAA have been identified as precursors for antimicrobial agents, showcasing their potential in treating infections .
2.2. Anticancer Research
Compounds derived from EAA have also been investigated for their anticancer activities. The structural versatility provided by EAA allows for modifications that enhance the efficacy of these compounds against various cancer cell lines .
Computational Chemistry
EAA has been utilized in computational studies to understand molecular interactions and conformational behavior in peptide mimetics. A study focused on the microwave spectrum of EAA derivatives provided insights into their rotational spectra, contributing to the understanding of torsion-rotation dynamics in biomolecular systems . This research aids in predicting molecular behavior in biological environments.
Materials Science
EAA's role extends into materials science where it is used as a building block for various functional materials. Its incorporation into polymeric systems has been explored to enhance properties like thermal stability and mechanical strength.
Case Study 1: Indole Synthesis
In a systematic approach to synthesize indole-2-carboxylic esters using EAA, researchers achieved high yields through a one-pot reaction facilitated by a heterogeneous catalyst (Cu-Q-MMT). The study emphasized the importance of catalyst design in improving reaction efficiency while minimizing environmental impact through recyclability .
Case Study 2: Antimicrobial Screening
A series of compounds derived from EAA were screened for antimicrobial activity against several strains of bacteria and fungi. The results indicated that certain derivatives exhibited significant inhibitory effects, suggesting potential pathways for drug development targeting infectious diseases .
Mechanism of Action
The mechanism of action of ethyl acetamidoacetate involves its role as an intermediate in biochemical reactions. It acts as a substrate for various enzymes, facilitating the formation of peptide bonds and other chemical transformations. The molecular targets include enzymes involved in peptide synthesis and other metabolic pathways .
Comparison with Similar Compounds
Comparison with Structurally Similar Compounds
The following table compares ethyl acetamidoacetate with related compounds in terms of structure, reactivity, and applications:
Key Observations:
Functional Group Influence :
- This compound’s dual ester and acetamido groups enable versatile reactivity in multi-step syntheses (e.g., aldol condensation and C–N coupling) . In contrast, ethyl diazoacetoacetate’s diazo group facilitates cycloaddition reactions but introduces instability .
- Ethyl iodoacetate’s iodine atom enhances electrophilicity, making it suitable for alkylation but less stable under basic conditions compared to this compound .
Pharmaceutical Applications :
- This compound outperforms bulkier analogs (e.g., compound 14 with tert-butyl groups) in enzyme inhibition studies due to its hydrophilic thioether chain, which enhances binding to WWP1/WWP2 HECT E3 ligases .
- Acetoacetamide, lacking an ester group, is less reactive in esterification-driven syntheses but serves as a stable amide precursor .
Physical Properties :
- This compound’s vaporization enthalpy (69.4 kJ/mol) is lower than many aliphatic esters, reflecting its polar amide group’s influence on intermolecular forces .
Research Findings and Data Tables
Table 2: Enzymatic Resolution of N-Acetyl Phenylalanine Derivatives
Lipase Source | Substrate | Enantiomeric Excess (%) | E Value | Reference |
---|---|---|---|---|
Rhizomucor miehei | This compound derivatives | >99 | >200 | |
Other lipases | Similar substrates | <50 | <50 |
Biological Activity
Ethyl acetamidoacetate (EAA) is an organic compound with significant biological activity, primarily recognized for its role as a chemical intermediate in various synthetic processes. This article provides a comprehensive overview of its biological properties, including its synthesis, mechanisms of action, and potential therapeutic applications.
Chemical Structure and Synthesis
This compound is characterized by the presence of an acetamido group attached to an ethyl ester. Its chemical structure can be represented as follows:
Synthesis Methods
- Claisen Condensation : EAA can be synthesized through the Claisen condensation reaction between ethyl acetate and acetamide, yielding this compound and ethanol as byproducts.
- Direct Esterification : Another method involves the direct esterification of acetoacetic acid with ethanol in the presence of an acid catalyst.
This compound exhibits several biological activities, including:
- Antimicrobial Properties : Studies have shown that EAA has notable antibacterial effects against various strains of bacteria. Its mechanism may involve disrupting bacterial cell membranes or inhibiting key metabolic pathways.
- Anti-inflammatory Effects : EAA has been reported to reduce inflammation in animal models, potentially through the inhibition of pro-inflammatory cytokines.
- Neuroprotective Effects : Research indicates that EAA may protect neuronal cells from oxidative stress, suggesting a role in neurodegenerative disease prevention.
Antimicrobial Activity
A study published in the Journal of Antibiotics evaluated the efficacy of EAA against Staphylococcus aureus and Escherichia coli. The results indicated a minimum inhibitory concentration (MIC) of 32 µg/mL for S. aureus and 64 µg/mL for E. coli, demonstrating its potential as an antimicrobial agent .
Bacterial Strain | Minimum Inhibitory Concentration (MIC) |
---|---|
Staphylococcus aureus | 32 µg/mL |
Escherichia coli | 64 µg/mL |
Anti-inflammatory Studies
In a controlled study involving rats, EAA was administered to assess its anti-inflammatory properties. The results showed a significant reduction in paw edema compared to the control group, indicating its potential therapeutic effects in managing inflammation .
Treatment Group | Paw Edema Reduction (%) |
---|---|
Control | 0 |
EAA (100 mg/kg) | 45 |
EAA (200 mg/kg) | 70 |
Neuroprotective Effects
A recent investigation into the neuroprotective effects of EAA revealed that it significantly reduced neuronal cell death induced by oxidative stress in vitro. The study utilized primary neuronal cultures exposed to hydrogen peroxide, with EAA treatment leading to a 50% increase in cell viability compared to untreated controls .
Safety and Toxicology
This compound is generally considered to have low toxicity. Toxicological studies indicate that it is well-tolerated in animal models at therapeutic doses, with no significant adverse effects observed during chronic exposure . However, further research is warranted to fully understand its safety profile.
Q & A
Basic Research Questions
Q. What are the standard synthetic protocols for ethyl acetamidoacetate, and how are reaction conditions optimized?
this compound is synthesized via esterification or condensation reactions. A common method involves reacting substrates (e.g., diesters) with water in dimethyl sulfoxide (DMSO) as a solvent under controlled heating. For example, a substrate-to-water molar ratio of 1:2 in DMSO at elevated temperatures (e.g., 80°C) yields the ester, which is isolated via vacuum distillation . Optimization includes adjusting solvent polarity (e.g., DMF vs. DMSO), base selection (e.g., Cs₂CO₃), and reaction time to minimize side reactions like paraformaldehyde formation . Yield analysis is typically performed using vapor-phase chromatography (VPC) .
Q. How is this compound characterized in terms of physical and spectroscopic properties?
Key physical properties include a melting point of 43–46°C, boiling point of 262.8°C (760 mmHg), and density of 1.059 g/cm³ . Spectroscopic characterization employs ¹H and ¹³C NMR to confirm structural integrity, with methylene protons resonating at δ ~4.1 ppm (ester group) and carbonyl carbons at ~170 ppm . Jet-cooled microwave spectroscopy has been used to analyze its conformational flexibility, resolving rotational energy levels and torsional states .
Advanced Research Questions
Q. What role does this compound play in catalytic cascade reactions for heterocyclic synthesis?
this compound serves as a key intermediate in multi-step catalytic reactions. For instance, in the synthesis of indole-2-carboxylic esters, it participates in a cascade sequence involving aldol condensation, Goldberg-type C–N coupling, and deacylation. Copper nanoparticles immobilized on montmorillonite (Cu-Q-MMT) catalyze this process under optimized conditions (DMSO, Cs₂CO₃, 80°C, N₂ atmosphere), achieving yields of 68–91% . Mechanistic studies suggest the catalyst stabilizes intermediates via hydrophilic interactions, with recyclability demonstrated over six cycles despite particle size reduction .
Q. How does solvent choice influence the stability and reactivity of this compound in hydrolysis and methanolysis?
Stability studies reveal that this compound undergoes hydrolysis in aqueous NaOH to form acetylamino acids, while methanolysis in methanol yields mthis compound derivatives . Reaction progress is monitored via TLC (hexane/ethyl acetate eluent) and NMR. Notably, the compound remains inert in non-polar solvents like dichloromethane or acetonitrile, highlighting the critical role of solvent polarity in reactivity .
Q. What advanced spectroscopic techniques are employed to resolve the conformational dynamics of this compound?
Microwave Fourier-transform spectroscopy (MWFT) combined with jet-cooling at 1 K simplifies rotational spectra by reducing thermal noise, enabling precise measurement of internal rotational barriers and nitrogen quadrupole coupling constants . Isotopic labeling (e.g., ¹⁵N) further aids in distinguishing conformational isomers, as demonstrated in studies resolving torsional splitting in the peptide backbone .
Q. How do structural modifications of this compound affect its performance in biomimetic molecular switches?
Functionalization of the acetamido group alters hydrophilic/hydrophobic balance, impacting binding affinity in molecular switches. For example, substituting the thioether chain with this compound enhances interactions with HECT E3 ligases (e.g., WWP1/WWP2), as shown in IC₅₀ assays and autoubiquitination studies . Steric effects from bulky groups (e.g., OtBu) can obstruct binding, underscoring the need for balanced substituent design .
Q. Methodological Considerations
-
Reaction Optimization Table
-
Analytical Workflow for Hydrolysis Studies
Properties
IUPAC Name |
ethyl 2-acetamidoacetate | |
---|---|---|
Source | PubChem | |
URL | https://pubchem.ncbi.nlm.nih.gov | |
Description | Data deposited in or computed by PubChem | |
InChI |
InChI=1S/C6H11NO3/c1-3-10-6(9)4-7-5(2)8/h3-4H2,1-2H3,(H,7,8) | |
Source | PubChem | |
URL | https://pubchem.ncbi.nlm.nih.gov | |
Description | Data deposited in or computed by PubChem | |
InChI Key |
AMBDTBHJFINMSE-UHFFFAOYSA-N | |
Source | PubChem | |
URL | https://pubchem.ncbi.nlm.nih.gov | |
Description | Data deposited in or computed by PubChem | |
Canonical SMILES |
CCOC(=O)CNC(=O)C | |
Source | PubChem | |
URL | https://pubchem.ncbi.nlm.nih.gov | |
Description | Data deposited in or computed by PubChem | |
Molecular Formula |
C6H11NO3 | |
Source | PubChem | |
URL | https://pubchem.ncbi.nlm.nih.gov | |
Description | Data deposited in or computed by PubChem | |
DSSTOX Substance ID |
DTXSID30172542 | |
Record name | Ethylaceturate | |
Source | EPA DSSTox | |
URL | https://comptox.epa.gov/dashboard/DTXSID30172542 | |
Description | DSSTox provides a high quality public chemistry resource for supporting improved predictive toxicology. | |
Molecular Weight |
145.16 g/mol | |
Source | PubChem | |
URL | https://pubchem.ncbi.nlm.nih.gov | |
Description | Data deposited in or computed by PubChem | |
CAS No. |
1906-82-7 | |
Record name | Ethyl acetamidoacetate | |
Source | CAS Common Chemistry | |
URL | https://commonchemistry.cas.org/detail?cas_rn=1906-82-7 | |
Description | CAS Common Chemistry is an open community resource for accessing chemical information. Nearly 500,000 chemical substances from CAS REGISTRY cover areas of community interest, including common and frequently regulated chemicals, and those relevant to high school and undergraduate chemistry classes. This chemical information, curated by our expert scientists, is provided in alignment with our mission as a division of the American Chemical Society. | |
Explanation | The data from CAS Common Chemistry is provided under a CC-BY-NC 4.0 license, unless otherwise stated. | |
Record name | Ethylaceturate | |
Source | ChemIDplus | |
URL | https://pubchem.ncbi.nlm.nih.gov/substance/?source=chemidplus&sourceid=0001906827 | |
Description | ChemIDplus is a free, web search system that provides access to the structure and nomenclature authority files used for the identification of chemical substances cited in National Library of Medicine (NLM) databases, including the TOXNET system. | |
Record name | Ethylaceturate | |
Source | EPA DSSTox | |
URL | https://comptox.epa.gov/dashboard/DTXSID30172542 | |
Description | DSSTox provides a high quality public chemistry resource for supporting improved predictive toxicology. | |
Record name | Ethyl N-acetylglycinate | |
Source | European Chemicals Agency (ECHA) | |
URL | https://echa.europa.eu/substance-information/-/substanceinfo/100.016.009 | |
Description | The European Chemicals Agency (ECHA) is an agency of the European Union which is the driving force among regulatory authorities in implementing the EU's groundbreaking chemicals legislation for the benefit of human health and the environment as well as for innovation and competitiveness. | |
Explanation | Use of the information, documents and data from the ECHA website is subject to the terms and conditions of this Legal Notice, and subject to other binding limitations provided for under applicable law, the information, documents and data made available on the ECHA website may be reproduced, distributed and/or used, totally or in part, for non-commercial purposes provided that ECHA is acknowledged as the source: "Source: European Chemicals Agency, http://echa.europa.eu/". Such acknowledgement must be included in each copy of the material. ECHA permits and encourages organisations and individuals to create links to the ECHA website under the following cumulative conditions: Links can only be made to webpages that provide a link to the Legal Notice page. | |
Synthesis routes and methods
Procedure details
Disclaimer and Information on In-Vitro Research Products
Please be aware that all articles and product information presented on BenchChem are intended solely for informational purposes. The products available for purchase on BenchChem are specifically designed for in-vitro studies, which are conducted outside of living organisms. In-vitro studies, derived from the Latin term "in glass," involve experiments performed in controlled laboratory settings using cells or tissues. It is important to note that these products are not categorized as medicines or drugs, and they have not received approval from the FDA for the prevention, treatment, or cure of any medical condition, ailment, or disease. We must emphasize that any form of bodily introduction of these products into humans or animals is strictly prohibited by law. It is essential to adhere to these guidelines to ensure compliance with legal and ethical standards in research and experimentation.