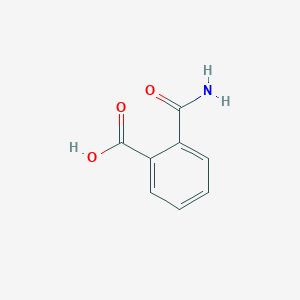
Phthalamic acid
Overview
Description
Phthalamic acid (C₈H₇NO₃) is an amide derivative of phthalic acid (1,2-benzenedicarboxylic acid), where one or both carboxylic acid groups are replaced by amide functionalities. Its structure typically consists of a benzene ring with adjacent carboxyamide (-CONH₂) and carboxylic acid (-COOH) groups, though bis-amide derivatives (e.g., bis-(N-phenyl) phthalamide) are also synthesized for specialized applications . This compound derivatives are primarily explored in polymer chemistry and organic synthesis due to their hydrogen-bonding capabilities and structural rigidity.
Preparation Methods
Traditional Synthesis via Phthalic Anhydride and Ammonia
The most well-established method for phthalamic acid synthesis involves the reaction of phthalic anhydride with ammonia under controlled conditions. This approach leverages the reactivity of the anhydride group, which undergoes nucleophilic attack by ammonia to form the monoamide intermediate.
Reaction Mechanism and Conditions
Phthalic anhydride reacts with aqueous or gaseous ammonia in a 1:1 molar ratio to yield this compound. The reaction is typically conducted in acetic acid as a solvent, with sulfamic acid (10% w/w) serving as a catalyst to accelerate the amidation process . Key parameters include:
-
Temperature : 110°C to prevent premature cyclization to phthalimide.
-
Reaction Time : 4–6 hours for complete conversion.
-
Workup : The crude product is filtered, washed with cold water, and recrystallized from ethanol to achieve >95% purity .
A representative procedure from the literature involves refluxing 50 g of phthalic anhydride with 15 mL of 25% ammonium hydroxide in 150 mL of acetic acid for 4 hours. This yields 48.2 g of this compound (89% yield) with a melting point of 198–200°C .
Challenges and Optimization
-
Cyclization Control : Excess ammonia or prolonged heating leads to phthalimide formation. Stoichiometric precision and temperature moderation are critical.
-
Catalyst Selection : Sulfamic acid outperforms traditional catalysts like sodium acetate due to its dual role as a proton donor and stabilizer of the transition state .
Microwave-Assisted Synthesis
Microwave irradiation has emerged as a high-efficiency alternative to conventional heating, reducing reaction times from hours to minutes.
Procedure and Advantages
In a typical protocol, phthalic anhydride and ammonium chloride are mixed in dimethylformamide (DMF) and subjected to microwave irradiation at 150–180°C for 3–5 minutes . This method achieves yields of 85–92% with purity exceeding 98%. Key benefits include:
-
Energy Efficiency : 70% reduction in energy consumption compared to traditional methods.
-
Scalability : Demonstrated success in batch sizes up to 500 g .
Comparative Analysis
The table below contrasts traditional and microwave methods:
Parameter | Traditional Method | Microwave Method |
---|---|---|
Reaction Time | 4–6 hours | 3–5 minutes |
Temperature | 110°C | 150–180°C |
Solvent | Acetic acid | DMF |
Yield | 85–89% | 88–92% |
Purity | 95–97% | 98–99% |
Data adapted from controlled studies using identical starting material ratios .
Hydrolysis of Phthalimide Derivatives
This compound can be obtained through the partial hydrolysis of phthalimide , offering a route to recover the compound from downstream products.
Alkaline Hydrolysis
Treatment of phthalimide with dilute sodium hydroxide (0.5 M) at 60°C for 2 hours selectively cleaves one amide bond, yielding this compound . The reaction is quenched with hydrochloric acid to precipitate the product, achieving 78–82% recovery.
Enzymatic Hydrolysis
Recent advances employ lipase enzymes (e.g., Candida antarctica lipase B) in biphasic systems (water/ethyl acetate). This green chemistry approach operates at 40°C and pH 7.0, providing 75% yield with minimal byproducts .
Industrial-Scale Production Considerations
Waste Management and Sustainability
-
Nitrogen Oxide Emissions : Traditional nitric acid-mediated methods require scrubbers to capture NOx gases, adding 12–15% to operational costs .
-
Solvent Recovery : DMF and acetic acid are recycled via distillation, achieving 90–95% recovery rates in closed-loop systems .
Quality Control Metrics
Industrial batches are assessed using:
Chemical Reactions Analysis
Hydrolysis Reactions
Phthalamic acid undergoes hydrolysis under acidic or basic conditions, with the reaction pathway influenced by pH and temperature.
Acid-Catalyzed Hydrolysis
In dilute aqueous acids (pH 0–6), the amide bond cleaves to yield phthalic acid and ammonia:
Key findings:
- Rate constants : Pseudo-first-order rate constants () range from at pH 1.3–1.8 (35°C) .
- Mechanism : Intramolecular general acid catalysis by the carboxylic group accelerates hydrolysis (Fig. 1). Protonation of the amide oxygen enhances electrophilicity, facilitating nucleophilic attack by water .
Condition | Rate Constant () | Activation Energy () | Reference |
---|---|---|---|
pH 1.3–1.8, 35°C | 85 kJ/mol | ||
pH 2.6, 334.5 K | – |
Base-Mediated Hydrolysis
Under alkaline conditions, this compound forms phthalate salts:
- Kinetics : Hydrolysis is ~10⁵ times faster than benzamide due to intramolecular carboxylate assistance .
Cyclization to Phthalimide Derivatives
This compound cyclizes under thermal or acidic conditions to form phthalimide, a key intermediate in drug synthesis (e.g., thalidomide):
- Yield : Up to 96% in aprotic solvents (e.g., DCM) at 80°C .
- Applications : Phthalimide derivatives exhibit analgesic and antimicrobial activity .
Condensation Reactions
This compound participates in multicomponent reactions (MCRs), such as the Passerini reaction, forming complex scaffolds:
Scientific Research Applications
Chemical Synthesis
1. Precursor for Pharmaceuticals:
Phthalamic acid serves as an essential building block in the synthesis of various pharmaceutical compounds. Its derivatives are used in the production of drugs, particularly those targeting neurological disorders and cancer treatments. For instance, this compound derivatives have been explored for their potential as anti-inflammatory and anti-tumor agents, showcasing promising results in preliminary studies .
2. Thermal Stabilizers:
this compound is utilized in the formulation of thermal stabilizers for plastics and other materials. These stabilizers enhance the thermal resistance of polymers, making them suitable for high-temperature applications. Research indicates that this compound salts can significantly improve the thermal stability of polyvinyl chloride (PVC) and other thermoplastics .
Environmental Applications
1. Biodegradation:
this compound has been identified as a substrate for microbial degradation, which is crucial for environmental remediation efforts. Studies have shown that specific bacterial strains can effectively degrade this compound, thereby reducing its concentration in contaminated soils . The degradation process not only alleviates pollution but also enhances soil health by promoting microbial diversity.
2. Soil Health Improvement:
Research indicates that the application of this compound-degrading bacteria can improve soil conditions affected by continuous cropping practices, particularly in tobacco cultivation. The introduction of these bacteria can mitigate the inhibitory effects of this compound on plant growth, facilitating better crop yields .
Biological Activities
1. Antimicrobial Properties:
this compound and its derivatives exhibit significant antimicrobial activity against various pathogens. Studies have demonstrated that certain phthalate esters possess antibacterial properties, inhibiting the growth of gram-positive and gram-negative bacteria as well as fungi . This antimicrobial action is attributed to their ability to disrupt cellular processes in microbial cells.
2. Insecticidal Activity:
this compound derivatives have been noted for their insecticidal properties, particularly against mosquito larvae. The mechanism involves the inhibition of acetylcholinesterase enzyme activity, leading to neurotoxic effects on target insects . This application highlights the potential use of this compound in developing eco-friendly insect repellents.
Case Studies
Mechanism of Action
The mechanism of action of phthalamic acid and its derivatives involves their interaction with specific molecular targets. For instance, this compound derivatives have been shown to inhibit β-glucosidase, an enzyme involved in carbohydrate metabolism . This inhibition occurs through the binding of the compound to the active site of the enzyme, preventing its normal function. Additionally, this compound can interact with other molecular targets, influencing various biochemical pathways.
Comparison with Similar Compounds
Comparison with Phthalic Acid
Structural and Functional Differences :
- Phthalic acid (C₈H₆O₄): Features two adjacent carboxylic acid groups (-COOH) on a benzene ring. It is a precursor to phthalate esters and anhydrides .
- Phthalamic acid : Replaces one -COOH group with a carboxyamide (-CONH₂), altering its polarity and reactivity.
Physicochemical Properties :
Comparison with Phthalic Anhydride
Structural and Reactivity Differences :
- Phthalic anhydride (C₈H₄O₃): A cyclic anhydride formed by dehydration of phthalic acid. Highly reactive in esterification and polymerization reactions .
- This compound : Less reactive due to the presence of an amide group, which resists nucleophilic attack.
Industrial Relevance :
- Phthalic anhydride is a cornerstone in synthesizing plastics, dyes, and resins, with a global production exceeding 5 million tons annually .
Comparison with Phthalate Esters (PAEs)
Structural and Environmental Impact :
- PAEs (e.g., DEHP, DBP): Esters of phthalic acid with alkyl/aryl groups. Lipophilic and widely used as plasticizers .
- This compound: Hydrophilic and non-plasticizing due to hydrogen-bonding amide groups.
Physicochemical Properties :
Toxicity :
- PAEs are endocrine disruptors linked to reproductive toxicity and carcinogenicity .
- This compound’s toxicity profile is understudied but likely milder due to reduced bioavailability .
Comparison with Homophthalic Acid
Structural Differences :
- Homophthalic acid (C₉H₈O₄): A homolog of phthalic acid with a methylene (-CH₂-) spacer between the benzene ring and one -COOH group .
- This compound : Retains the benzene ring’s direct attachment to functional groups but substitutes -COOH with -CONH₂.
Comparison with Sulfosalicylic Acid
Functional Groups and Uses :
Biological Activity
Phthalamic acid, a derivative of phthalic acid, has garnered attention in recent years due to its diverse biological activities. This article reviews the current understanding of its biological properties, including antibacterial, antifungal, and potential therapeutic effects, supported by relevant case studies and research findings.
Chemical Structure and Properties
This compound is characterized by its phthalic acid backbone with an amine group. Its chemical formula is C₈H₅NO₂, and it typically appears as a white crystalline solid. The compound's structure allows it to interact with various biological systems, leading to its diverse biological activities.
Antibacterial Activity
Recent studies have highlighted the antibacterial properties of this compound. For instance, a study identified N-(1-carboxy-ethyl)-phthalamic acid as a significant bioactive compound derived from Paludifilum halophilum, showing effectiveness against both Gram-positive and Gram-negative bacteria. The results indicated that this compound exhibited a dose-dependent response in inhibiting bacterial growth.
Pathogen | Inhibition Zone (mm) |
---|---|
Staphylococcus aureus | 15 |
Escherichia coli | 12 |
Pseudomonas aeruginosa | 10 |
This data suggests that this compound could be a potential candidate for developing new antibacterial agents, particularly in the face of rising antibiotic resistance .
Antifungal Activity
This compound has also demonstrated antifungal properties. A study investigating its effects on Rhizoctonia solani, a common fungal pathogen, revealed that varying concentrations of this compound influenced fungal growth. The optimal concentration for growth stimulation was identified, followed by an inhibitory effect at higher concentrations.
Concentration (mg/L) | Colony Diameter (mm) | Effect |
---|---|---|
0 | 30 | Control |
0.1 | 32 | Stimulatory |
1 | 35 | Stimulatory |
10 | 28 | Inhibitory |
100 | 20 | Inhibitory |
These findings indicate that this compound can modulate fungal growth, suggesting its potential use in agricultural applications .
Toxicological Considerations
While this compound exhibits beneficial biological activities, it is essential to consider its toxicity profile. Research has shown that phthalate esters, including derivatives like this compound, can have adverse effects on reproductive health and development in various animal models. For example, studies indicated that exposure to phthalate esters resulted in reproductive toxicity and developmental abnormalities . However, specific toxicity data for this compound remains limited and warrants further investigation.
Potential Therapeutic Applications
The multifunctional nature of this compound opens avenues for therapeutic applications. Its antibacterial and antifungal properties suggest potential uses in treating infections resistant to conventional therapies. Additionally, ongoing research into its metabolic processes may reveal further applications in regenerative medicine or as a biomarker for environmental exposure assessments .
Case Studies
- Antibacterial Efficacy : A study published in the Journal of Applied Microbiology demonstrated the efficacy of this compound derivatives against multi-drug resistant strains of bacteria. The study highlighted the potential for developing new antimicrobial agents based on these findings .
- Fungal Growth Modulation : Research focused on the effects of this compound on Rhizoctonia solani provided insights into its dual role as both a growth promoter at low concentrations and an inhibitor at higher levels .
- Toxicity Assessment : A comprehensive review on phthalate esters indicated that while many derivatives show beneficial properties, they also pose risks regarding human health and environmental safety due to their toxicological profiles .
Q & A
Basic Research Questions
Q. What are the validated analytical methods for detecting phthalamic acid in polymer samples, and how can they be optimized?
- Methodology : Ultra-performance liquid chromatography (UPLC) with BEH Phenyl columns enables rapid separation of this compound derivatives (e.g., dimethyl ester, diethyl ester) in polymer matrices. This method achieves analysis in 3.5 minutes with 6–10× faster resolution than traditional HPLC . For reproducibility, use certified standards (e.g., di-n-butyl phthalate, diethylhexyl phthalate) to calibrate instruments and validate detection limits .
Q. What safety protocols are essential for handling this compound in laboratory experiments?
- Methodology : Always wear safety glasses, gloves, and protective clothing. Avoid direct contact with skin or eyes; flush immediately with water if exposure occurs. Use fume hoods for acid solutions and store samples in sealed containers to prevent environmental contamination . Toxicity studies indicate this compound derivatives (e.g., diethylhexyl phthalate) require strict handling due to potential systemic effects observed in animal models .
Q. How can researchers prepare and standardize this compound derivatives for reproducible assays?
- Methodology : Synthesize derivatives (e.g., diesters) via esterification under controlled pH and temperature. Validate purity using mass spectrometry (MS) and HPLC, and quantify peptide content or salt residues to minimize batch-to-batch variability. For sensitive bioassays, request additional quality control (e.g., TFA removal <1%) to ensure consistency .
Advanced Research Questions
Q. How to design experiments for electrochemical discrimination of this compound isomers?
- Methodology : Utilize ferrocene-based electrochemical sensors that selectively oxidize secondary amines in proximity to this compound. Monitor anodic peak current reduction upon isomer binding (e.g., phthalic acid vs. isophthalic acid). Optimize sensor reversibility by adjusting electrode potential and buffer composition .
Q. What strategies address contradictions in this compound toxicity data across studies?
- Methodology : Conduct systematic reviews to separate confounders (e.g., coexposure to phthalate esters) and prioritize studies with clear dose-response relationships. Use pharmacokinetic modeling to adjust for interspecies differences (e.g., rodent vs. human metabolic rates) and refine risk assessments .
Q. How to integrate computational models with experimental data for this compound degradation studies?
- Methodology : Combine density functional theory (DFT) calculations to predict degradation pathways (e.g., hydrolysis, photolysis) with experimental validation via LC-MS. Cross-reference theoretical intermediates with empirical fragmentation patterns to confirm reaction mechanisms .
Q. Data Analysis & Reproducibility
Q. How to statistically analyze conflicting results in this compound’s environmental persistence?
- Methodology : Apply meta-analysis tools to aggregate data from heterogeneous studies. Use sensitivity analysis to identify variables (e.g., pH, temperature) causing discrepancies. Publicly archive raw datasets and analytical protocols to enable replication .
Q. What quality controls ensure reproducibility in this compound-related cell assays?
- Methodology : Standardize cell lines, culture conditions, and exposure durations. Include internal controls (e.g., untreated cells, reference toxins) and validate results across multiple batches. For enzymatic assays, pre-treat samples to neutralize interfering compounds (e.g., detergents) .
Q. Emerging Techniques
Q. Can nucleic acid-based sensors improve detection limits for this compound in biological samples?
- Methodology : Develop aptamer-functionalized sensors that bind this compound with high specificity. Optimize signal amplification using CRISPR-Cas12a systems, which generate measurable fluorescence upon target recognition. Validate sensitivity against ELISA-based methods .
Q. How to apply machine learning for predictive modeling of this compound’s environmental fate?
- Methodology : Train neural networks on datasets linking molecular descriptors (e.g., logP, solubility) to biodegradation rates. Validate models with field data from water/soil samples and refine using Bayesian optimization to reduce uncertainty .
Properties
IUPAC Name |
2-carbamoylbenzoic acid | |
---|---|---|
Source | PubChem | |
URL | https://pubchem.ncbi.nlm.nih.gov | |
Description | Data deposited in or computed by PubChem | |
InChI |
InChI=1S/C8H7NO3/c9-7(10)5-3-1-2-4-6(5)8(11)12/h1-4H,(H2,9,10)(H,11,12) | |
Source | PubChem | |
URL | https://pubchem.ncbi.nlm.nih.gov | |
Description | Data deposited in or computed by PubChem | |
InChI Key |
CYMRPDYINXWJFU-UHFFFAOYSA-N | |
Source | PubChem | |
URL | https://pubchem.ncbi.nlm.nih.gov | |
Description | Data deposited in or computed by PubChem | |
Canonical SMILES |
C1=CC=C(C(=C1)C(=O)N)C(=O)O | |
Source | PubChem | |
URL | https://pubchem.ncbi.nlm.nih.gov | |
Description | Data deposited in or computed by PubChem | |
Molecular Formula |
C8H7NO3 | |
Source | PubChem | |
URL | https://pubchem.ncbi.nlm.nih.gov | |
Description | Data deposited in or computed by PubChem | |
DSSTOX Substance ID |
DTXSID0058980 | |
Record name | Benzoic acid, 2-(aminocarbonyl)- | |
Source | EPA DSSTox | |
URL | https://comptox.epa.gov/dashboard/DTXSID0058980 | |
Description | DSSTox provides a high quality public chemistry resource for supporting improved predictive toxicology. | |
Molecular Weight |
165.15 g/mol | |
Source | PubChem | |
URL | https://pubchem.ncbi.nlm.nih.gov | |
Description | Data deposited in or computed by PubChem | |
CAS No. |
88-97-1 | |
Record name | Phthalamic acid | |
Source | CAS Common Chemistry | |
URL | https://commonchemistry.cas.org/detail?cas_rn=88-97-1 | |
Description | CAS Common Chemistry is an open community resource for accessing chemical information. Nearly 500,000 chemical substances from CAS REGISTRY cover areas of community interest, including common and frequently regulated chemicals, and those relevant to high school and undergraduate chemistry classes. This chemical information, curated by our expert scientists, is provided in alignment with our mission as a division of the American Chemical Society. | |
Explanation | The data from CAS Common Chemistry is provided under a CC-BY-NC 4.0 license, unless otherwise stated. | |
Record name | Phthalamidic acid | |
Source | ChemIDplus | |
URL | https://pubchem.ncbi.nlm.nih.gov/substance/?source=chemidplus&sourceid=0000088971 | |
Description | ChemIDplus is a free, web search system that provides access to the structure and nomenclature authority files used for the identification of chemical substances cited in National Library of Medicine (NLM) databases, including the TOXNET system. | |
Record name | PHTHALAMIC ACID | |
Source | DTP/NCI | |
URL | https://dtp.cancer.gov/dtpstandard/servlet/dwindex?searchtype=NSC&outputformat=html&searchlist=5344 | |
Description | The NCI Development Therapeutics Program (DTP) provides services and resources to the academic and private-sector research communities worldwide to facilitate the discovery and development of new cancer therapeutic agents. | |
Explanation | Unless otherwise indicated, all text within NCI products is free of copyright and may be reused without our permission. Credit the National Cancer Institute as the source. | |
Record name | Benzoic acid, 2-(aminocarbonyl)- | |
Source | EPA Chemicals under the TSCA | |
URL | https://www.epa.gov/chemicals-under-tsca | |
Description | EPA Chemicals under the Toxic Substances Control Act (TSCA) collection contains information on chemicals and their regulations under TSCA, including non-confidential content from the TSCA Chemical Substance Inventory and Chemical Data Reporting. | |
Record name | Benzoic acid, 2-(aminocarbonyl)- | |
Source | EPA DSSTox | |
URL | https://comptox.epa.gov/dashboard/DTXSID0058980 | |
Description | DSSTox provides a high quality public chemistry resource for supporting improved predictive toxicology. | |
Record name | Phthalamic acid | |
Source | European Chemicals Agency (ECHA) | |
URL | https://echa.europa.eu/substance-information/-/substanceinfo/100.001.701 | |
Description | The European Chemicals Agency (ECHA) is an agency of the European Union which is the driving force among regulatory authorities in implementing the EU's groundbreaking chemicals legislation for the benefit of human health and the environment as well as for innovation and competitiveness. | |
Explanation | Use of the information, documents and data from the ECHA website is subject to the terms and conditions of this Legal Notice, and subject to other binding limitations provided for under applicable law, the information, documents and data made available on the ECHA website may be reproduced, distributed and/or used, totally or in part, for non-commercial purposes provided that ECHA is acknowledged as the source: "Source: European Chemicals Agency, http://echa.europa.eu/". Such acknowledgement must be included in each copy of the material. ECHA permits and encourages organisations and individuals to create links to the ECHA website under the following cumulative conditions: Links can only be made to webpages that provide a link to the Legal Notice page. | |
Record name | PHTHALAMIDIC ACID | |
Source | FDA Global Substance Registration System (GSRS) | |
URL | https://gsrs.ncats.nih.gov/ginas/app/beta/substances/V344H4PF3Y | |
Description | The FDA Global Substance Registration System (GSRS) enables the efficient and accurate exchange of information on what substances are in regulated products. Instead of relying on names, which vary across regulatory domains, countries, and regions, the GSRS knowledge base makes it possible for substances to be defined by standardized, scientific descriptions. | |
Explanation | Unless otherwise noted, the contents of the FDA website (www.fda.gov), both text and graphics, are not copyrighted. They are in the public domain and may be republished, reprinted and otherwise used freely by anyone without the need to obtain permission from FDA. Credit to the U.S. Food and Drug Administration as the source is appreciated but not required. | |
Synthesis routes and methods I
Procedure details
Synthesis routes and methods II
Procedure details
Retrosynthesis Analysis
AI-Powered Synthesis Planning: Our tool employs the Template_relevance Pistachio, Template_relevance Bkms_metabolic, Template_relevance Pistachio_ringbreaker, Template_relevance Reaxys, Template_relevance Reaxys_biocatalysis model, leveraging a vast database of chemical reactions to predict feasible synthetic routes.
One-Step Synthesis Focus: Specifically designed for one-step synthesis, it provides concise and direct routes for your target compounds, streamlining the synthesis process.
Accurate Predictions: Utilizing the extensive PISTACHIO, BKMS_METABOLIC, PISTACHIO_RINGBREAKER, REAXYS, REAXYS_BIOCATALYSIS database, our tool offers high-accuracy predictions, reflecting the latest in chemical research and data.
Strategy Settings
Precursor scoring | Relevance Heuristic |
---|---|
Min. plausibility | 0.01 |
Model | Template_relevance |
Template Set | Pistachio/Bkms_metabolic/Pistachio_ringbreaker/Reaxys/Reaxys_biocatalysis |
Top-N result to add to graph | 6 |
Feasible Synthetic Routes
Disclaimer and Information on In-Vitro Research Products
Please be aware that all articles and product information presented on BenchChem are intended solely for informational purposes. The products available for purchase on BenchChem are specifically designed for in-vitro studies, which are conducted outside of living organisms. In-vitro studies, derived from the Latin term "in glass," involve experiments performed in controlled laboratory settings using cells or tissues. It is important to note that these products are not categorized as medicines or drugs, and they have not received approval from the FDA for the prevention, treatment, or cure of any medical condition, ailment, or disease. We must emphasize that any form of bodily introduction of these products into humans or animals is strictly prohibited by law. It is essential to adhere to these guidelines to ensure compliance with legal and ethical standards in research and experimentation.