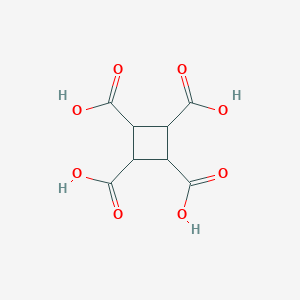
1,2,3,4-Cyclobutanetetracarboxylic acid
Overview
Description
Norcamphor, also known as bicyclo[2.2.1]heptan-2-one, is an organic compound classified as a bicyclic ketone. It is an analog of camphor but lacks the three methyl groups present in camphor. Norcamphor is a colorless solid at room temperature and is commonly used as a building block in organic synthesis .
Mechanism of Action
Target of Action
1,2,3,4-Cyclobutanetetracarboxylic acid primarily targets metal ions, particularly cobalt (II) ions . The compound forms a complex with these ions under hydrothermal conditions . This interaction plays a crucial role in the compound’s function and its applications in various fields.
Mode of Action
The compound interacts with its targets by forming a complex. Specifically, it acts as a capping ligand in the preparation of gold colloids . This interaction results in changes to the structure of the targeted ions, influencing their properties and behaviors .
Biochemical Pathways
It’s known that the compound can form complexes with alkali metal ions . These complexes have been synthesized and crystallographically characterized, indicating that the compound may influence the properties and behaviors of these ions .
Pharmacokinetics
It’s soluble in methanol , which suggests that it may have good bioavailability when administered in suitable formulations.
Result of Action
The molecular and cellular effects of this compound’s action largely depend on its role as a capping ligand in the preparation of gold colloids . The formation of these colloids can influence the properties of the targeted ions, potentially leading to changes at the molecular and cellular levels .
Action Environment
The action, efficacy, and stability of this compound can be influenced by various environmental factors. For instance, the compound forms a complex with cobalt (II) ions under hydrothermal conditions . This suggests that temperature and pressure may play a role in its function. Additionally, the compound’s solubility in methanol indicates that the presence of certain solvents can also affect its action and stability.
Preparation Methods
Synthetic Routes and Reaction Conditions: Norcamphor is typically synthesized from norbornene. The process involves the formation of 2-exo-norbornyl formate, which is then oxidized to produce norcamphor. The detailed steps are as follows :
-
Formation of 2-exo-Norbornyl Formate:
- Norbornene is reacted with formic acid under reflux conditions for about 4 hours.
- The resulting solution is distilled to remove excess formic acid, yielding 2-exo-norbornyl formate.
-
Oxidation to Norcamphor:
- The 2-exo-norbornyl formate is dissolved in acetone and cooled with an ice bath.
- Chromic acid solution is added dropwise to the mixture, maintaining the reaction temperature between 20-30°C.
- The reaction mixture is stirred overnight, and the product is purified through distillation.
Industrial Production Methods: Industrial production of norcamphor follows similar synthetic routes but on a larger scale, with optimized reaction conditions to maximize yield and purity.
Chemical Reactions Analysis
Types of Reactions: Norcamphor undergoes various chemical reactions, including oxidation, reduction, and substitution :
-
Oxidation:
- Norcamphor can be hydroxylated by cytochrome P450 enzymes, resulting in the formation of hydroxylated products at different carbon positions.
-
Reduction:
- Norcamphor can be reduced to norborneol using reducing agents such as sodium borohydride.
-
Substitution:
- Norcamphor can undergo substitution reactions, where the carbonyl group is replaced by other functional groups.
Common Reagents and Conditions:
Oxidation: Chromic acid, cytochrome P450 enzymes.
Reduction: Sodium borohydride.
Substitution: Various nucleophiles depending on the desired product.
Major Products:
- Hydroxylated norcamphor derivatives.
- Norborneol.
- Substituted norcamphor derivatives.
Scientific Research Applications
Norcamphor has several applications in scientific research :
-
Chemistry:
- Used as a building block in organic synthesis.
- Precursor to norborneols and other bicyclic compounds.
-
Biology:
- Studied for its interactions with enzymes such as cytochrome P450.
-
Medicine:
- Potential applications in drug development due to its structural similarity to camphor.
-
Industry:
- Used in the production of polymers and other advanced materials.
Comparison with Similar Compounds
- Camphor (bicyclo[2.2.1]heptan-2-one with three methyl groups).
- Borneol (a bicyclic alcohol derived from camphor).
- Fenchone (a bicyclic ketone similar to camphor).
Norcamphor’s unique structure makes it a valuable compound in various fields of research and industry, offering distinct advantages over its analogs.
Properties
IUPAC Name |
cyclobutane-1,2,3,4-tetracarboxylic acid | |
---|---|---|
Source | PubChem | |
URL | https://pubchem.ncbi.nlm.nih.gov | |
Description | Data deposited in or computed by PubChem | |
InChI |
InChI=1S/C8H8O8/c9-5(10)1-2(6(11)12)4(8(15)16)3(1)7(13)14/h1-4H,(H,9,10)(H,11,12)(H,13,14)(H,15,16) | |
Source | PubChem | |
URL | https://pubchem.ncbi.nlm.nih.gov | |
Description | Data deposited in or computed by PubChem | |
InChI Key |
CURBACXRQKTCKZ-UHFFFAOYSA-N | |
Source | PubChem | |
URL | https://pubchem.ncbi.nlm.nih.gov | |
Description | Data deposited in or computed by PubChem | |
Canonical SMILES |
C1(C(C(C1C(=O)O)C(=O)O)C(=O)O)C(=O)O | |
Source | PubChem | |
URL | https://pubchem.ncbi.nlm.nih.gov | |
Description | Data deposited in or computed by PubChem | |
Molecular Formula |
C8H8O8 | |
Source | PubChem | |
URL | https://pubchem.ncbi.nlm.nih.gov | |
Description | Data deposited in or computed by PubChem | |
DSSTOX Substance ID |
DTXSID20967681 | |
Record name | Cyclobutane-1,2,3,4-tetracarboxylic acid | |
Source | EPA DSSTox | |
URL | https://comptox.epa.gov/dashboard/DTXSID20967681 | |
Description | DSSTox provides a high quality public chemistry resource for supporting improved predictive toxicology. | |
Molecular Weight |
232.14 g/mol | |
Source | PubChem | |
URL | https://pubchem.ncbi.nlm.nih.gov | |
Description | Data deposited in or computed by PubChem | |
CAS No. |
53159-92-5, 720-21-8 | |
Record name | NSC 131453 | |
Source | ChemIDplus | |
URL | https://pubchem.ncbi.nlm.nih.gov/substance/?source=chemidplus&sourceid=0053159925 | |
Description | ChemIDplus is a free, web search system that provides access to the structure and nomenclature authority files used for the identification of chemical substances cited in National Library of Medicine (NLM) databases, including the TOXNET system. | |
Record name | 53159-92-5 | |
Source | DTP/NCI | |
URL | https://dtp.cancer.gov/dtpstandard/servlet/dwindex?searchtype=NSC&outputformat=html&searchlist=131453 | |
Description | The NCI Development Therapeutics Program (DTP) provides services and resources to the academic and private-sector research communities worldwide to facilitate the discovery and development of new cancer therapeutic agents. | |
Explanation | Unless otherwise indicated, all text within NCI products is free of copyright and may be reused without our permission. Credit the National Cancer Institute as the source. | |
Record name | NSC102999 | |
Source | DTP/NCI | |
URL | https://dtp.cancer.gov/dtpstandard/servlet/dwindex?searchtype=NSC&outputformat=html&searchlist=102999 | |
Description | The NCI Development Therapeutics Program (DTP) provides services and resources to the academic and private-sector research communities worldwide to facilitate the discovery and development of new cancer therapeutic agents. | |
Explanation | Unless otherwise indicated, all text within NCI products is free of copyright and may be reused without our permission. Credit the National Cancer Institute as the source. | |
Record name | Cyclobutane-1,2,3,4-tetracarboxylic acid | |
Source | EPA DSSTox | |
URL | https://comptox.epa.gov/dashboard/DTXSID20967681 | |
Description | DSSTox provides a high quality public chemistry resource for supporting improved predictive toxicology. | |
Record name | 1,2,3,4-Cyclobutanetetracarboxylic Acid | |
Source | European Chemicals Agency (ECHA) | |
URL | https://echa.europa.eu/information-on-chemicals | |
Description | The European Chemicals Agency (ECHA) is an agency of the European Union which is the driving force among regulatory authorities in implementing the EU's groundbreaking chemicals legislation for the benefit of human health and the environment as well as for innovation and competitiveness. | |
Explanation | Use of the information, documents and data from the ECHA website is subject to the terms and conditions of this Legal Notice, and subject to other binding limitations provided for under applicable law, the information, documents and data made available on the ECHA website may be reproduced, distributed and/or used, totally or in part, for non-commercial purposes provided that ECHA is acknowledged as the source: "Source: European Chemicals Agency, http://echa.europa.eu/". Such acknowledgement must be included in each copy of the material. ECHA permits and encourages organisations and individuals to create links to the ECHA website under the following cumulative conditions: Links can only be made to webpages that provide a link to the Legal Notice page. | |
Synthesis routes and methods
Procedure details
Retrosynthesis Analysis
AI-Powered Synthesis Planning: Our tool employs the Template_relevance Pistachio, Template_relevance Bkms_metabolic, Template_relevance Pistachio_ringbreaker, Template_relevance Reaxys, Template_relevance Reaxys_biocatalysis model, leveraging a vast database of chemical reactions to predict feasible synthetic routes.
One-Step Synthesis Focus: Specifically designed for one-step synthesis, it provides concise and direct routes for your target compounds, streamlining the synthesis process.
Accurate Predictions: Utilizing the extensive PISTACHIO, BKMS_METABOLIC, PISTACHIO_RINGBREAKER, REAXYS, REAXYS_BIOCATALYSIS database, our tool offers high-accuracy predictions, reflecting the latest in chemical research and data.
Strategy Settings
Precursor scoring | Relevance Heuristic |
---|---|
Min. plausibility | 0.01 |
Model | Template_relevance |
Template Set | Pistachio/Bkms_metabolic/Pistachio_ringbreaker/Reaxys/Reaxys_biocatalysis |
Top-N result to add to graph | 6 |
Feasible Synthetic Routes
Disclaimer and Information on In-Vitro Research Products
Please be aware that all articles and product information presented on BenchChem are intended solely for informational purposes. The products available for purchase on BenchChem are specifically designed for in-vitro studies, which are conducted outside of living organisms. In-vitro studies, derived from the Latin term "in glass," involve experiments performed in controlled laboratory settings using cells or tissues. It is important to note that these products are not categorized as medicines or drugs, and they have not received approval from the FDA for the prevention, treatment, or cure of any medical condition, ailment, or disease. We must emphasize that any form of bodily introduction of these products into humans or animals is strictly prohibited by law. It is essential to adhere to these guidelines to ensure compliance with legal and ethical standards in research and experimentation.