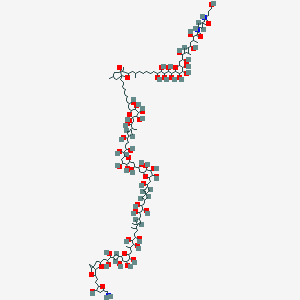
Palytoxin from palythoa
- Click on QUICK INQUIRY to receive a quote from our team of experts.
- With the quality product at a COMPETITIVE price, you can focus more on your research.
Overview
Description
Palytoxin is a highly toxic compound originally isolated from the marine zoanthid Palythoa toxica. It is one of the most potent non-protein toxins known, with a complex structure that includes a long carbon chain and multiple hydroxyl groups. Palytoxin is known for its intense vasoconstrictive properties and its ability to disrupt cellular ion gradients .
Preparation Methods
Synthetic Routes and Reaction Conditions
The synthesis of palytoxin is extremely challenging due to its complex structure, which includes 64 stereocenters and numerous functional groups. The first total synthesis was achieved by Yoshito Kishi and his team in 1994.
Industrial Production Methods
Industrial production of palytoxin is not feasible due to its complex structure and the difficulty of its synthesis. Instead, it is typically extracted from natural sources, such as the zoanthid corals of the genus Palythoa .
Chemical Reactions Analysis
Types of Reactions
Palytoxin undergoes various chemical reactions, including:
Oxidation: Introduction of hydroxyl groups.
Reduction: Reduction of double bonds.
Substitution: Replacement of functional groups.
Common Reagents and Conditions
Common reagents used in the synthesis and modification of palytoxin include oxidizing agents like osmium tetroxide and reducing agents such as lithium aluminum hydride. Reaction conditions often involve precise temperature control and the use of protective groups to ensure selectivity .
Major Products
The major products formed from these reactions are typically intermediates in the synthesis of palytoxin, including various polyether and hydroxylated compounds .
Scientific Research Applications
Palytoxin has several scientific research applications:
Chemistry: Used as a model compound for studying complex natural product synthesis.
Biology: Employed in studies of ion channels and cellular ion gradients.
Medicine: Investigated for its potential use in cancer therapy due to its ability to disrupt cellular functions.
Industry: Limited use due to its high toxicity, but it serves as a valuable tool in biochemical research
Mechanism of Action
Palytoxin exerts its effects by binding to and modifying the function of the sodium-potassium ATPase pump. This binding converts the pump into a non-selective cation channel, leading to the disruption of ion gradients across the cell membrane. This disruption causes cell depolarization and can lead to cell death .
Comparison with Similar Compounds
Similar Compounds
Maitotoxin: Another highly toxic marine toxin with a different mechanism of action.
Tetrodotoxin: A potent neurotoxin that blocks sodium channels.
Saxitoxin: A neurotoxin that also targets sodium channels.
Uniqueness
Palytoxin is unique due to its complex structure and its specific mechanism of action on the sodium-potassium ATPase pump. Unlike other marine toxins, it converts this pump into a cation channel, leading to a unique set of physiological effects .
If you have any more questions or need further details, feel free to ask!
Properties
CAS No. |
77734-91-9 |
---|---|
Molecular Formula |
C129H223N3O54 |
Molecular Weight |
2680.1 g/mol |
IUPAC Name |
(E,2S,3R,5R,8R,9S)-10-[(2R,3R,4R,5S,6R)-6-[(1S,2R,3S,4S,5R,11S)-12-[(1R,3S,5S,7R)-5-[(8S)-9-[(2R,3R,4R,5R,6S)-6-[(E,2S,3S,6S,9R,10R)-10-[(2S,4R,5S,6R)-6-[(2R,3R)-4-[(2R,3S,4R,5R,6S)-6-[(2S,3Z,5E,8R,9S,10R,12Z,17S,18R,19R,20R)-21-[(2R,3R,4R,5S,6R)-6-[(Z,3R,4R)-5-[(1S,3R,5R,7R)-7-[2-[(2R,3R,5S)-5-(aminomethyl)-3-hydroxyoxolan-2-yl]ethyl]-2,6-dioxabicyclo[3.2.1]octan-3-yl]-3,4-dihydroxypent-1-enyl]-3,4,5-trihydroxyoxan-2-yl]-2,8,9,10,17,18,19-heptahydroxy-20-methyl-14-methylidenehenicosa-3,5,12-trienyl]-3,4,5-trihydroxyoxan-2-yl]-2,3-dihydroxybutyl]-4,5-dihydroxyoxan-2-yl]-2,6,9,10-tetrahydroxy-3-methyldec-4-enyl]-3,4,5,6-tetrahydroxyoxan-2-yl]-8-hydroxynonyl]-1,3-dimethyl-6,8-dioxabicyclo[3.2.1]octan-7-yl]-1,2,3,4,5-pentahydroxy-11-methyldodecyl]-3,4,5-trihydroxyoxan-2-yl]-2,5,8,9-tetrahydroxy-N-[(E)-3-(3-hydroxypropylamino)-3-oxoprop-1-enyl]-3,7-dimethyldec-6-enamide |
InChI |
InChI=1S/C129H223N3O54/c1-62(29-33-81(143)108(158)103(153)68(7)47-93-111(161)117(167)110(160)91(180-93)36-35-76(138)82(144)51-73-50-74-53-92(178-73)90(177-74)38-37-89-85(147)52-75(61-130)179-89)23-20-28-78(140)105(155)77(139)26-18-13-16-25-70(135)48-94-112(162)118(168)113(163)97(181-94)55-84(146)83(145)54-95-107(157)87(149)57-96(182-95)106(156)80(142)34-32-69(134)31-30-65(4)88(150)60-129(176)125(174)123(173)115(165)99(184-129)49-71(136)24-15-10-9-11-19-40-128-59-64(3)58-127(8,186-128)100(185-128)44-63(2)22-14-12-17-27-79(141)109(159)116(166)120(170)122(172)124-121(171)119(169)114(164)98(183-124)56-86(148)102(152)66(5)45-72(137)46-67(6)104(154)126(175)132-42-39-101(151)131-41-21-43-133/h13,16,18,20,23,25,30-31,35-36,39,42,45,63-65,67-100,102-125,133-150,152-174,176H,1,9-12,14-15,17,19,21-22,24,26-29,32-34,37-38,40-41,43-44,46-61,130H2,2-8H3,(H,131,151)(H,132,175)/b18-13+,23-20-,25-16-,31-30+,36-35-,42-39+,66-45+/t63-,64-,65-,67+,68+,69+,70+,71-,72-,73-,74+,75-,76+,77+,78+,79+,80+,81-,82+,83+,84+,85+,86-,87+,88-,89+,90+,91+,92-,93+,94-,95+,96-,97+,98+,99+,100+,102+,103+,104-,105-,106+,107-,108+,109-,110+,111-,112-,113+,114-,115-,116-,117-,118+,119+,120+,121-,122-,123+,124-,125+,127+,128-,129-/m0/s1 |
InChI Key |
CWODDUGJZSCNGB-HQNRRURTSA-N |
SMILES |
CC1CC2(C(OC(C1)(O2)CCCCCCCC(CC3C(C(C(C(O3)(CC(C(C)C=CC(CCC(C(C4CC(C(C(O4)CC(C(CC5C(C(C(C(O5)CC(C=CC=CCC(C(C(CC=CC(=C)CCC(C(C(C(C)CC6C(C(C(C(O6)C=CC(C(CC7CC8CC(O7)C(O8)CCC9C(CC(O9)CN)O)O)O)O)O)O)O)O)O)O)O)O)O)O)O)O)O)O)O)O)O)O)O)O)O)O)O)O)O)CC(C)CCCCCC(C(C(C(C(C1C(C(C(C(O1)CC(C(C(=CC(CC(C)C(C(=O)NC=CC(=O)NCCCO)O)O)C)O)O)O)O)O)O)O)O)O)O)C |
Isomeric SMILES |
C[C@H]1C[C@@]2([C@H](O[C@](C1)(O2)CCCCCCC[C@@H](C[C@@H]3[C@@H]([C@H]([C@H]([C@@](O3)(C[C@@H]([C@@H](C)/C=C/[C@H](CC[C@H]([C@H]([C@@H]4C[C@H]([C@@H]([C@H](O4)C[C@H]([C@@H](C[C@@H]5[C@H]([C@@H]([C@H]([C@@H](O5)C[C@@H](/C=C\C=C\C[C@H]([C@@H]([C@@H](C/C=C\C(=C)CC[C@@H]([C@H]([C@@H]([C@H](C)C[C@@H]6[C@@H]([C@H]([C@@H]([C@H](O6)/C=C\[C@H]([C@@H](C[C@@H]7C[C@@H]8C[C@H](O7)[C@H](O8)CC[C@@H]9[C@@H](C[C@H](O9)CN)O)O)O)O)O)O)O)O)O)O)O)O)O)O)O)O)O)O)O)O)O)O)O)O)O)O)O)O)O)C[C@@H](C)CCCCC[C@H]([C@@H]([C@@H]([C@H]([C@@H]([C@@H]1[C@H]([C@@H]([C@H]([C@H](O1)C[C@@H]([C@@H](/C(=C/[C@@H](C[C@@H](C)[C@@H](C(=O)N/C=C/C(=O)NCCCO)O)O)/C)O)O)O)O)O)O)O)O)O)O)C |
Canonical SMILES |
CC1CC2(C(OC(C1)(O2)CCCCCCCC(CC3C(C(C(C(O3)(CC(C(C)C=CC(CCC(C(C4CC(C(C(O4)CC(C(CC5C(C(C(C(O5)CC(C=CC=CCC(C(C(CC=CC(=C)CCC(C(C(C(C)CC6C(C(C(C(O6)C=CC(C(CC7CC8CC(O7)C(O8)CCC9C(CC(O9)CN)O)O)O)O)O)O)O)O)O)O)O)O)O)O)O)O)O)O)O)O)O)O)O)O)O)O)O)O)O)CC(C)CCCCCC(C(C(C(C(C1C(C(C(C(O1)CC(C(C(=CC(CC(C)C(C(=O)NC=CC(=O)NCCCO)O)O)C)O)O)O)O)O)O)O)O)O)O)C |
Color/Form |
Non-crystalline /solid/ |
solubility |
Water soluble substance |
Synonyms |
Palytoxin from Palythoa tuberculosa |
Origin of Product |
United States |
Disclaimer and Information on In-Vitro Research Products
Please be aware that all articles and product information presented on BenchChem are intended solely for informational purposes. The products available for purchase on BenchChem are specifically designed for in-vitro studies, which are conducted outside of living organisms. In-vitro studies, derived from the Latin term "in glass," involve experiments performed in controlled laboratory settings using cells or tissues. It is important to note that these products are not categorized as medicines or drugs, and they have not received approval from the FDA for the prevention, treatment, or cure of any medical condition, ailment, or disease. We must emphasize that any form of bodily introduction of these products into humans or animals is strictly prohibited by law. It is essential to adhere to these guidelines to ensure compliance with legal and ethical standards in research and experimentation.