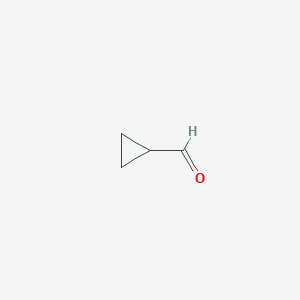
Cyclopropanecarboxaldehyde
Overview
Description
Cyclopropanecarboxaldehyde is an organic compound with the molecular formula C₄H₆O. It is a colorless liquid with a pungent odor and is known for its reactivity due to the strained three-membered cyclopropane ring. This compound is used as an intermediate in organic synthesis and has applications in various fields, including pharmaceuticals and agrochemicals .
Preparation Methods
Synthetic Routes and Reaction Conditions: Cyclopropanecarboxaldehyde can be synthesized through several methods:
Reduction of Cyclopropanecarboxylic Acid: One common method involves the reduction of cyclopropanecarboxylic acid using reducing agents such as lithium aluminum hydride.
Ring Contraction of Cyclobutane Derivatives: Another method involves the ring contraction of cyclobutane derivatives, such as the thermal isomerization of 2,3-dihydrofuran under superatmospheric pressure.
Oxidation of Cyclopropanemethanol: Cyclopropanemethanol can be oxidized using pyridinium chlorochromate in dichloromethane to yield this compound.
Industrial Production Methods: Industrial production of this compound often involves the thermal isomerization of 2,3-dihydrofuran at high temperatures and pressures. This method is efficient and yields high purity this compound .
Chemical Reactions Analysis
Cyclopropanecarboxaldehyde undergoes various chemical reactions, including:
Oxidation: It can be oxidized to cyclopropanecarboxylic acid using oxidizing agents such as potassium permanganate.
Reduction: Reduction with reagents like lithium aluminum hydride yields cyclopropanemethanol.
Substitution: It can undergo nucleophilic substitution reactions, where the aldehyde group is replaced by other functional groups.
Common Reagents and Conditions:
Oxidizing Agents: Potassium permanganate, chromium trioxide.
Reducing Agents: Lithium aluminum hydride, sodium borohydride.
Catalysts: Palladium complexes for cycloaddition reactions.
Major Products:
Cyclopropanecarboxylic Acid: From oxidation.
Cyclopropanemethanol: From reduction.
Cyclopentene Derivatives: From cycloaddition reactions.
Scientific Research Applications
Cyclopropanecarboxaldehyde has several scientific research applications:
Organic Synthesis: It is used as an intermediate in the synthesis of various organic compounds, including pharmaceuticals and agrochemicals.
Medicinal Chemistry: It is involved in the synthesis of potential anti-diabetic agents and cytochrome P450 inhibitors.
Material Science: It is used in the preparation of nickel complexes and other coordination compounds.
Biological Studies: It serves as a building block for the synthesis of biologically active molecules.
Mechanism of Action
The mechanism of action of cyclopropanecarboxaldehyde involves its reactivity due to the strained cyclopropane ring and the aldehyde functional group. The cyclopropane ring can undergo ring-opening reactions, while the aldehyde group can participate in nucleophilic addition and substitution reactions. These properties make it a versatile intermediate in organic synthesis .
Comparison with Similar Compounds
- Cyclopentanecarboxaldehyde
- Cyclohexanecarboxaldehyde
- Cyclopropanemethanol
- Cyclopropylcarboxylic acid
Properties
IUPAC Name |
cyclopropanecarbaldehyde | |
---|---|---|
Source | PubChem | |
URL | https://pubchem.ncbi.nlm.nih.gov | |
Description | Data deposited in or computed by PubChem | |
InChI |
InChI=1S/C4H6O/c5-3-4-1-2-4/h3-4H,1-2H2 | |
Source | PubChem | |
URL | https://pubchem.ncbi.nlm.nih.gov | |
Description | Data deposited in or computed by PubChem | |
InChI Key |
JMYVMOUINOAAPA-UHFFFAOYSA-N | |
Source | PubChem | |
URL | https://pubchem.ncbi.nlm.nih.gov | |
Description | Data deposited in or computed by PubChem | |
Canonical SMILES |
C1CC1C=O | |
Source | PubChem | |
URL | https://pubchem.ncbi.nlm.nih.gov | |
Description | Data deposited in or computed by PubChem | |
Molecular Formula |
C4H6O | |
Source | PubChem | |
URL | https://pubchem.ncbi.nlm.nih.gov | |
Description | Data deposited in or computed by PubChem | |
DSSTOX Substance ID |
DTXSID0051736 | |
Record name | Cyclopropanecarboxaldehyde | |
Source | EPA DSSTox | |
URL | https://comptox.epa.gov/dashboard/DTXSID0051736 | |
Description | DSSTox provides a high quality public chemistry resource for supporting improved predictive toxicology. | |
Molecular Weight |
70.09 g/mol | |
Source | PubChem | |
URL | https://pubchem.ncbi.nlm.nih.gov | |
Description | Data deposited in or computed by PubChem | |
Physical Description |
Liquid | |
Record name | Cyclopropanecarboxaldehyde | |
Source | EPA Chemicals under the TSCA | |
URL | https://www.epa.gov/chemicals-under-tsca | |
Description | EPA Chemicals under the Toxic Substances Control Act (TSCA) collection contains information on chemicals and their regulations under TSCA, including non-confidential content from the TSCA Chemical Substance Inventory and Chemical Data Reporting. | |
CAS No. |
1489-69-6 | |
Record name | Cyclopropanecarboxaldehyde | |
Source | CAS Common Chemistry | |
URL | https://commonchemistry.cas.org/detail?cas_rn=1489-69-6 | |
Description | CAS Common Chemistry is an open community resource for accessing chemical information. Nearly 500,000 chemical substances from CAS REGISTRY cover areas of community interest, including common and frequently regulated chemicals, and those relevant to high school and undergraduate chemistry classes. This chemical information, curated by our expert scientists, is provided in alignment with our mission as a division of the American Chemical Society. | |
Explanation | The data from CAS Common Chemistry is provided under a CC-BY-NC 4.0 license, unless otherwise stated. | |
Record name | Cyclopropanecarboxaldehyde | |
Source | ChemIDplus | |
URL | https://pubchem.ncbi.nlm.nih.gov/substance/?source=chemidplus&sourceid=0001489696 | |
Description | ChemIDplus is a free, web search system that provides access to the structure and nomenclature authority files used for the identification of chemical substances cited in National Library of Medicine (NLM) databases, including the TOXNET system. | |
Record name | Cyclopropanecarboxaldehyde | |
Source | EPA Chemicals under the TSCA | |
URL | https://www.epa.gov/chemicals-under-tsca | |
Description | EPA Chemicals under the Toxic Substances Control Act (TSCA) collection contains information on chemicals and their regulations under TSCA, including non-confidential content from the TSCA Chemical Substance Inventory and Chemical Data Reporting. | |
Record name | Cyclopropanecarboxaldehyde | |
Source | EPA DSSTox | |
URL | https://comptox.epa.gov/dashboard/DTXSID0051736 | |
Description | DSSTox provides a high quality public chemistry resource for supporting improved predictive toxicology. | |
Record name | Cyclopropanecarboxaldehyde | |
Source | European Chemicals Agency (ECHA) | |
URL | https://echa.europa.eu/information-on-chemicals | |
Description | The European Chemicals Agency (ECHA) is an agency of the European Union which is the driving force among regulatory authorities in implementing the EU's groundbreaking chemicals legislation for the benefit of human health and the environment as well as for innovation and competitiveness. | |
Explanation | Use of the information, documents and data from the ECHA website is subject to the terms and conditions of this Legal Notice, and subject to other binding limitations provided for under applicable law, the information, documents and data made available on the ECHA website may be reproduced, distributed and/or used, totally or in part, for non-commercial purposes provided that ECHA is acknowledged as the source: "Source: European Chemicals Agency, http://echa.europa.eu/". Such acknowledgement must be included in each copy of the material. ECHA permits and encourages organisations and individuals to create links to the ECHA website under the following cumulative conditions: Links can only be made to webpages that provide a link to the Legal Notice page. | |
Synthesis routes and methods I
Procedure details
Synthesis routes and methods II
Procedure details
Retrosynthesis Analysis
AI-Powered Synthesis Planning: Our tool employs the Template_relevance Pistachio, Template_relevance Bkms_metabolic, Template_relevance Pistachio_ringbreaker, Template_relevance Reaxys, Template_relevance Reaxys_biocatalysis model, leveraging a vast database of chemical reactions to predict feasible synthetic routes.
One-Step Synthesis Focus: Specifically designed for one-step synthesis, it provides concise and direct routes for your target compounds, streamlining the synthesis process.
Accurate Predictions: Utilizing the extensive PISTACHIO, BKMS_METABOLIC, PISTACHIO_RINGBREAKER, REAXYS, REAXYS_BIOCATALYSIS database, our tool offers high-accuracy predictions, reflecting the latest in chemical research and data.
Strategy Settings
Precursor scoring | Relevance Heuristic |
---|---|
Min. plausibility | 0.01 |
Model | Template_relevance |
Template Set | Pistachio/Bkms_metabolic/Pistachio_ringbreaker/Reaxys/Reaxys_biocatalysis |
Top-N result to add to graph | 6 |
Feasible Synthetic Routes
Q1: What is the molecular formula and weight of cyclopropanecarboxaldehyde (CPCA)?
A1: The molecular formula of this compound is C4H6O, and its molecular weight is 70.09 g/mol.
Q2: What are the key spectroscopic characteristics of CPCA?
A2: CPCA has been extensively studied using various spectroscopic techniques, including infrared (IR) [, ], Raman [], and microwave spectroscopy [, , , , , ]. These studies have provided insights into its vibrational modes, conformational stability, and rotational parameters.
Q3: CPCA exists in two isomeric forms. How do their spectroscopic properties differ?
A3: CPCA exists as cis and trans isomers, each exhibiting distinct spectroscopic signatures. Microwave spectroscopy has been particularly useful in characterizing the rotational spectra and dipole moments of both isomers []. The trans isomer, for instance, shows a strong absorption band in the infrared spectrum, which has been reassigned based on computational studies [].
Q4: Which conformer of CPCA is more stable and how is this influenced by the environment?
A4: While computational studies often predict the anti conformer to be more stable [], experimental findings regarding the relative stability of syn and anti-CPCA are contradictory and depend heavily on factors such as zero-point vibrational correction []. The stability order can be influenced by the surrounding environment, with the anti-conformer being favored in condensed phases [].
Q5: How has computational chemistry been employed to study CPCA?
A5: Computational methods such as DFT B3LYP [] and ab initio calculations [, ] have been employed to investigate various aspects of CPCA. These include the study of rotational isomerism [, ], conformational stability [], and vibrational frequencies for spectral assignment [].
Q6: What are some methods for synthesizing CPCA?
A6: CPCA can be synthesized through various methods. One approach involves the MacFadyen-Stevens reduction of cyclopropanecarboxylic acid []. Another method utilizes the oxidation of cyclopropanemethanol, either using cerium(IV) [] or through a Swern oxidation process [].
Q7: How does the Swern oxidation of specific cyclopropane derivatives lead to the formation of dihydrooxepines?
A7: Swern oxidation of 2-alkenyl-substituted 2-siloxy-1-(hydroxymethyl)cyclopropanes leads to the formation of 2,5-dihydrooxepines []. This transformation likely involves cyclopropanecarboxaldehydes as intermediates, undergoing a [, ]-sigmatropic rearrangement to form the seven-membered heterocycles [].
Q8: Can CPCA act as a precursor for other cyclic compounds?
A8: Yes, CPCA can serve as a precursor for various cyclic compounds. For example, it can be used in the synthesis of spirovetivane-type sesquiterpenoids via the thermal rearrangement of enol silyl ethers derived from 2-(cyclopropylmethylene)cycloalkanones [].
Q9: How has CPCA been utilized in the synthesis of fluorinated benzo(j)fluoranthene derivatives?
A9: CPCA derivatives have played a crucial role in synthesizing fluorinated benzo(j)fluoranthene compounds [, ]. One method involves the cyclodehydration of cyclopropanecarboxaldehydes [, ], offering a new synthetic route to these specific fluoranthene derivatives.
Q10: What are the applications of cyclopropylcarbinols derived from CPCA?
A10: Cyclopropylcarbinols, synthesized from CPCA, have found applications as intermediates in the stereospecific synthesis of (E)-homoallylic bromides []. This method improves the accessibility of these valuable compounds for various synthetic applications.
Q11: How can nickel catalysis be employed in reactions involving CPCA?
A11: Nickel catalysis, specifically using [Ni(cod)2] (cod = 1,5-cyclooctadiene) with different phosphine ligands, has proven valuable in reactions involving CPCA and its derivatives []. For instance, nickel-catalyzed defluorinative alkylation allows for the coupling of CPCA with trifluoromethyl alkenes []. Moreover, nickel complexes can mediate [3+2] cycloaddition reactions of CPCA derivatives with enones, leading to the formation of cyclopentane derivatives []. These methodologies highlight the versatility of CPCA in constructing complex molecular structures.
Q12: What is the significance of studying the dissociation pathways of molecules like 2,3-dihydrofuran, and how does it relate to CPCA?
A13: Studying the dissociation pathways of molecules like 2,3-dihydrofuran provides valuable insights into complex chemical reactions occurring in various environments, including combustion and astrochemistry []. The identification of CPCA as one of the dissociation products of 2,3-dihydrofuran using chirped-pulse Fourier transform microwave spectroscopy suggests its potential presence in such environments [].
Disclaimer and Information on In-Vitro Research Products
Please be aware that all articles and product information presented on BenchChem are intended solely for informational purposes. The products available for purchase on BenchChem are specifically designed for in-vitro studies, which are conducted outside of living organisms. In-vitro studies, derived from the Latin term "in glass," involve experiments performed in controlled laboratory settings using cells or tissues. It is important to note that these products are not categorized as medicines or drugs, and they have not received approval from the FDA for the prevention, treatment, or cure of any medical condition, ailment, or disease. We must emphasize that any form of bodily introduction of these products into humans or animals is strictly prohibited by law. It is essential to adhere to these guidelines to ensure compliance with legal and ethical standards in research and experimentation.