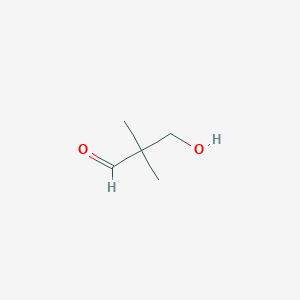
3-Hydroxy-2,2-dimethylpropanal
Overview
Description
It is a white to off-white solid with a melting point of 30-33°C and a boiling point of approximately 131.45°C . This compound is notable for its use in the synthesis of various chemical intermediates and its applications in organic synthesis.
Preparation Methods
Synthetic Routes and Reaction Conditions: 3-Hydroxy-2,2-dimethylpropanal is typically synthesized through the condensation of formaldehyde and isobutyraldehyde. The reaction is catalyzed by dibutyltin oxide, although calcium chloride or sodium carbonate can also be used as catalysts . The reaction proceeds under mild conditions, typically at room temperature, and yields the desired product after purification.
Industrial Production Methods: In industrial settings, the production of this compound involves similar synthetic routes but on a larger scale. The reaction conditions are optimized to maximize yield and purity, often involving continuous flow reactors and advanced purification techniques to ensure the product meets industrial standards .
Chemical Reactions Analysis
Types of Reactions: 3-Hydroxy-2,2-dimethylpropanal undergoes various chemical reactions, including:
Oxidation: It can be oxidized to form 3-hydroxy-2,2-dimethylpropanoic acid.
Reduction: Reduction reactions can convert it to 3-hydroxy-2,2-dimethylpropanol.
Substitution: It can participate in nucleophilic substitution reactions to form various derivatives.
Common Reagents and Conditions:
Oxidation: Common oxidizing agents include potassium permanganate and chromium trioxide.
Reduction: Sodium borohydride and lithium aluminum hydride are frequently used reducing agents.
Substitution: Reagents such as alkyl halides and acyl chlorides are used under basic or acidic conditions.
Major Products:
Oxidation: 3-Hydroxy-2,2-dimethylpropanoic acid.
Reduction: 3-Hydroxy-2,2-dimethylpropanol.
Substitution: Various substituted derivatives depending on the reagents used.
Scientific Research Applications
Synthesis and Mechanism of Action
3-Hydroxy-2,2-dimethylpropanal can be synthesized through the hydroxymethylation of isobutyraldehyde in the presence of a base such as potassium carbonate. Its mechanism of action involves its ability to participate in various chemical reactions due to the presence of hydroxyl and aldehyde functional groups, making it a valuable intermediate in organic synthesis.
Chemistry
This compound serves as an important intermediate in the synthesis of complex organic molecules, including pharmaceuticals and agrochemicals. It can be utilized for:
- Oxidation to produce 3-hydroxy-2,2-dimethylpropanoic acid.
- Reduction to yield 3-hydroxy-2,2-dimethylpropanol.
- Substitution reactions leading to various derivatives depending on the reagents used .
Biology
In biological research, this compound is used as a building block for synthesizing biologically active compounds such as enzyme inhibitors and receptor modulators. It has been involved in the preparation of chiral cyanohydrins which are crucial for developing therapeutic agents .
Medicine
Recent studies have explored the potential of this compound in treating metabolic disorders and cancer. For instance, derivatives synthesized from this compound have shown selective inhibition of colon cancer cell proliferation with IC values ranging from 0.12 mg/mL to 0.81 mg/mL . The compounds demonstrated specific action on cancerous cells without affecting normal cells, suggesting a targeted therapeutic potential .
Industry
In industrial applications, this compound is utilized for producing polymers and resins. Its role as a precursor in the synthesis of fine chemicals further highlights its versatility in various manufacturing processes .
Data Table: Summary of Applications
Application Area | Specific Use Cases | Notable Findings |
---|---|---|
Chemistry | Intermediate for pharmaceuticals | Used in oxidation and reduction reactions |
Biology | Building block for enzyme inhibitors | Important for synthesizing chiral cyanohydrins |
Medicine | Potential therapeutic agent | Inhibits colon cancer cell proliferation (IC = 0.12 - 0.81 mg/mL) |
Industry | Production of polymers and resins | Key precursor in fine chemical synthesis |
Case Studies
-
Colon Cancer Research :
A recent study focused on synthesizing derivatives from methyl-3-(4-chlorophenyl)-3-hydroxy-2,2-dimethylpropanoate that selectively inhibit colon cancer cell proliferation. The study found that certain compounds exhibited significant apoptotic activity against HCT-116 cells while sparing non-cancerous HEK-293 cells, indicating their potential for targeted cancer therapy . -
Synthesis of Biologically Active Compounds :
Research demonstrated that modifications of this compound led to the development of new compounds with enhanced biological activity against various targets, showcasing its utility in drug discovery and development processes .
Mechanism of Action
The mechanism of action of 3-hydroxy-2,2-dimethylpropanal involves its ability to participate in various chemical reactions due to the presence of both hydroxyl and aldehyde functional groups. These functional groups allow it to act as a versatile intermediate in organic synthesis, facilitating the formation of a wide range of products. The molecular targets and pathways involved depend on the specific reactions and applications being studied .
Comparison with Similar Compounds
- 2,2-Dimethyl-3-hydroxypropanoic acid
- 2,2-Dimethyl-3-hydroxypropionaldehyde
- 2,2-Dimethyl-3-hydroxypropyl acetate
Comparison: 3-Hydroxy-2,2-dimethylpropanal is unique due to its specific combination of hydroxyl and aldehyde functional groups, which provide it with distinct reactivity compared to similar compounds. For instance, while 2,2-dimethyl-3-hydroxypropanoic acid primarily undergoes reactions typical of carboxylic acids, this compound can participate in both aldehyde and alcohol chemistry, making it more versatile in synthetic applications .
Biological Activity
3-Hydroxy-2,2-dimethylpropanal, a compound with the chemical formula CHO, is notable for its diverse biological activities and applications in organic synthesis. This article explores its biological properties, mechanisms of action, and potential therapeutic applications based on current research findings.
This compound is characterized by its hydroxyl and aldehyde functional groups, which contribute to its reactivity in various chemical reactions. The compound exists as a white to off-white solid with a melting point of 30-33°C and a boiling point of approximately 131.45°C. Its structure allows it to participate in oxidation, reduction, and substitution reactions, making it a versatile intermediate in organic synthesis.
The biological activity of this compound is largely attributed to its ability to act as a substrate for various enzymatic reactions. The presence of the hydroxyl group enables it to form hydrogen bonds with biological macromolecules, influencing interactions with enzymes and receptors. This property is crucial for its role in synthesizing biologically active compounds, including enzyme inhibitors and receptor modulators .
Anticancer Properties
Recent studies have highlighted the anticancer potential of derivatives synthesized from this compound. For example, a series of compounds derived from this precursor exhibited selective inhibition of colon cancer cell proliferation. The IC values for these compounds ranged from 0.12 mg/mL to 0.81 mg/mL against HCT-116 cells, indicating significant antiproliferative activity . Notably, two specific derivatives demonstrated the highest inhibitory activity at an IC of 0.12 mg/mL.
Table 1: Anticancer Activity of Derivatives
Compound | IC (mg/mL) | Cell Line |
---|---|---|
Compound 7a | 0.12 | HCT-116 |
Compound 7g | 0.12 | HCT-116 |
Compound 7d | 0.69 | HeLa |
Doxorubicin | 2.29 | HeLa |
This data suggests that derivatives based on this compound may serve as lead compounds for developing new anticancer agents .
Enzyme Inhibition
Research has also focused on the role of this compound in inhibiting histone deacetylases (HDACs), which are critical targets in cancer therapy. Some derivatives have shown promising results as HDAC inhibitors (HDACIs), which can modulate gene expression and induce apoptosis in cancer cells . The structure-activity relationship (SAR) studies indicate that modifications to the core structure can enhance inhibitory potency.
Toxicological Profile
While exploring the biological activities of this compound and its derivatives, it is essential to consider their toxicological profiles. Preliminary assessments suggest that these compounds do not exhibit significant toxicity at therapeutic concentrations; however, comprehensive toxicological evaluations are necessary to establish safety profiles for potential clinical applications .
Case Studies
Several case studies have been conducted to investigate the biological effects of this compound:
- Colon Cancer Study : A study involving the synthesis of methyl esters derived from this compound demonstrated their ability to inhibit the proliferation of colon cancer cells significantly. The research highlighted the importance of structural modifications in enhancing anticancer activity.
- HDAC Inhibition : Another investigation focused on the synthesis of various amides and esters from this compound showed promising HDAC inhibitory activity, suggesting potential applications in epigenetic therapy for cancer treatment .
Properties
IUPAC Name |
3-hydroxy-2,2-dimethylpropanal | |
---|---|---|
Source | PubChem | |
URL | https://pubchem.ncbi.nlm.nih.gov | |
Description | Data deposited in or computed by PubChem | |
InChI |
InChI=1S/C5H10O2/c1-5(2,3-6)4-7/h3,7H,4H2,1-2H3 | |
Source | PubChem | |
URL | https://pubchem.ncbi.nlm.nih.gov | |
Description | Data deposited in or computed by PubChem | |
InChI Key |
JJMOMMLADQPZNY-UHFFFAOYSA-N | |
Source | PubChem | |
URL | https://pubchem.ncbi.nlm.nih.gov | |
Description | Data deposited in or computed by PubChem | |
Canonical SMILES |
CC(C)(CO)C=O | |
Source | PubChem | |
URL | https://pubchem.ncbi.nlm.nih.gov | |
Description | Data deposited in or computed by PubChem | |
Molecular Formula |
C5H10O2 | |
Source | PubChem | |
URL | https://pubchem.ncbi.nlm.nih.gov | |
Description | Data deposited in or computed by PubChem | |
DSSTOX Substance ID |
DTXSID9027231 | |
Record name | Propanal, 3-hydroxy-2,2-dimethyl- | |
Source | EPA DSSTox | |
URL | https://comptox.epa.gov/dashboard/DTXSID9027231 | |
Description | DSSTox provides a high quality public chemistry resource for supporting improved predictive toxicology. | |
Molecular Weight |
102.13 g/mol | |
Source | PubChem | |
URL | https://pubchem.ncbi.nlm.nih.gov | |
Description | Data deposited in or computed by PubChem | |
Physical Description |
Liquid; mp = 30-33 deg C; [MSDSonline] | |
Record name | 3-Hydroxy-2,2-dimethylpropionaldehyde | |
Source | Haz-Map, Information on Hazardous Chemicals and Occupational Diseases | |
URL | https://haz-map.com/Agents/3233 | |
Description | Haz-Map® is an occupational health database designed for health and safety professionals and for consumers seeking information about the adverse effects of workplace exposures to chemical and biological agents. | |
Explanation | Copyright (c) 2022 Haz-Map(R). All rights reserved. Unless otherwise indicated, all materials from Haz-Map are copyrighted by Haz-Map(R). No part of these materials, either text or image may be used for any purpose other than for personal use. Therefore, reproduction, modification, storage in a retrieval system or retransmission, in any form or by any means, electronic, mechanical or otherwise, for reasons other than personal use, is strictly prohibited without prior written permission. | |
CAS No. |
597-31-9, 31091-91-5 | |
Record name | Hydroxypivalaldehyde | |
Source | CAS Common Chemistry | |
URL | https://commonchemistry.cas.org/detail?cas_rn=597-31-9 | |
Description | CAS Common Chemistry is an open community resource for accessing chemical information. Nearly 500,000 chemical substances from CAS REGISTRY cover areas of community interest, including common and frequently regulated chemicals, and those relevant to high school and undergraduate chemistry classes. This chemical information, curated by our expert scientists, is provided in alignment with our mission as a division of the American Chemical Society. | |
Explanation | The data from CAS Common Chemistry is provided under a CC-BY-NC 4.0 license, unless otherwise stated. | |
Record name | 3-Hydroxy-2,2-dimethylpropionaldehyde | |
Source | ChemIDplus | |
URL | https://pubchem.ncbi.nlm.nih.gov/substance/?source=chemidplus&sourceid=0000597319 | |
Description | ChemIDplus is a free, web search system that provides access to the structure and nomenclature authority files used for the identification of chemical substances cited in National Library of Medicine (NLM) databases, including the TOXNET system. | |
Record name | NSC91821 | |
Source | DTP/NCI | |
URL | https://dtp.cancer.gov/dtpstandard/servlet/dwindex?searchtype=NSC&outputformat=html&searchlist=91821 | |
Description | The NCI Development Therapeutics Program (DTP) provides services and resources to the academic and private-sector research communities worldwide to facilitate the discovery and development of new cancer therapeutic agents. | |
Explanation | Unless otherwise indicated, all text within NCI products is free of copyright and may be reused without our permission. Credit the National Cancer Institute as the source. | |
Record name | Propanal, 3-hydroxy-2,2-dimethyl- | |
Source | EPA Chemicals under the TSCA | |
URL | https://www.epa.gov/chemicals-under-tsca | |
Description | EPA Chemicals under the Toxic Substances Control Act (TSCA) collection contains information on chemicals and their regulations under TSCA, including non-confidential content from the TSCA Chemical Substance Inventory and Chemical Data Reporting. | |
Record name | Propanal, 3-hydroxy-2,2-dimethyl- | |
Source | EPA DSSTox | |
URL | https://comptox.epa.gov/dashboard/DTXSID9027231 | |
Description | DSSTox provides a high quality public chemistry resource for supporting improved predictive toxicology. | |
Record name | 3-hydroxy-2,2-dimethylpropionaldehyde | |
Source | European Chemicals Agency (ECHA) | |
URL | https://echa.europa.eu/substance-information/-/substanceinfo/100.008.998 | |
Description | The European Chemicals Agency (ECHA) is an agency of the European Union which is the driving force among regulatory authorities in implementing the EU's groundbreaking chemicals legislation for the benefit of human health and the environment as well as for innovation and competitiveness. | |
Explanation | Use of the information, documents and data from the ECHA website is subject to the terms and conditions of this Legal Notice, and subject to other binding limitations provided for under applicable law, the information, documents and data made available on the ECHA website may be reproduced, distributed and/or used, totally or in part, for non-commercial purposes provided that ECHA is acknowledged as the source: "Source: European Chemicals Agency, http://echa.europa.eu/". Such acknowledgement must be included in each copy of the material. ECHA permits and encourages organisations and individuals to create links to the ECHA website under the following cumulative conditions: Links can only be made to webpages that provide a link to the Legal Notice page. | |
Record name | 3-HYDROXY-2,2-DIMETHYLPROPANAL | |
Source | FDA Global Substance Registration System (GSRS) | |
URL | https://gsrs.ncats.nih.gov/ginas/app/beta/substances/6DK0IR9LLR | |
Description | The FDA Global Substance Registration System (GSRS) enables the efficient and accurate exchange of information on what substances are in regulated products. Instead of relying on names, which vary across regulatory domains, countries, and regions, the GSRS knowledge base makes it possible for substances to be defined by standardized, scientific descriptions. | |
Explanation | Unless otherwise noted, the contents of the FDA website (www.fda.gov), both text and graphics, are not copyrighted. They are in the public domain and may be republished, reprinted and otherwise used freely by anyone without the need to obtain permission from FDA. Credit to the U.S. Food and Drug Administration as the source is appreciated but not required. | |
Record name | 3-HYDROXY-2,2-DIMETHYLPROPIONALDEHYDE | |
Source | Hazardous Substances Data Bank (HSDB) | |
URL | https://pubchem.ncbi.nlm.nih.gov/source/hsdb/5711 | |
Description | The Hazardous Substances Data Bank (HSDB) is a toxicology database that focuses on the toxicology of potentially hazardous chemicals. It provides information on human exposure, industrial hygiene, emergency handling procedures, environmental fate, regulatory requirements, nanomaterials, and related areas. The information in HSDB has been assessed by a Scientific Review Panel. | |
Retrosynthesis Analysis
AI-Powered Synthesis Planning: Our tool employs the Template_relevance Pistachio, Template_relevance Bkms_metabolic, Template_relevance Pistachio_ringbreaker, Template_relevance Reaxys, Template_relevance Reaxys_biocatalysis model, leveraging a vast database of chemical reactions to predict feasible synthetic routes.
One-Step Synthesis Focus: Specifically designed for one-step synthesis, it provides concise and direct routes for your target compounds, streamlining the synthesis process.
Accurate Predictions: Utilizing the extensive PISTACHIO, BKMS_METABOLIC, PISTACHIO_RINGBREAKER, REAXYS, REAXYS_BIOCATALYSIS database, our tool offers high-accuracy predictions, reflecting the latest in chemical research and data.
Strategy Settings
Precursor scoring | Relevance Heuristic |
---|---|
Min. plausibility | 0.01 |
Model | Template_relevance |
Template Set | Pistachio/Bkms_metabolic/Pistachio_ringbreaker/Reaxys/Reaxys_biocatalysis |
Top-N result to add to graph | 6 |
Feasible Synthetic Routes
Q1: What is unique about the structure of 3-hydroxy-2,2-dimethylpropanal?
A1: this compound exhibits an intriguing equilibrium between its monomeric and dimeric forms. In its crystalline state, the compound exists as a substituted 1,3-dioxane dimer, adopting a cis chair conformation. [] This dimer was confirmed through X-ray analysis, revealing two independent molecules within the asymmetric unit, each residing in separate layers with distinct hydrogen-bonding patterns. [] Upon melting, the dimer undergoes a reversible decomposition into the monomeric this compound. [] This process is akin to an anhydrous acid-catalyzed acetal hydrolysis, initiated by an intermolecular hydrogen atom transfer facilitated by hydrogen bonding. []
Q2: How can aqueous solutions of this compound be concentrated?
A2: A specific method has been patented for concentrating aqueous solutions of this compound. [, ] This method involves adding an entrainer, chosen from a group including 1-butanol, 2-butanol, 2-methyl-1-propanol, 1-pentanol, or 1-hexanol, to the aqueous solution containing this compound and/or its dimer. [] The resulting mixture undergoes azeotropic distillation using a distillation column, effectively removing water and the entrainer, thereby concentrating the desired compound. []
Q3: Can this compound be used to synthesize other compounds?
A3: Yes, this compound serves as a valuable precursor in organic synthesis. One example is the synthesis of dimethylmethylenebis(iminonitroxide) diradical. This diradical, with interesting magnetic properties, was successfully synthesized from this compound in a five-step process. []
Q4: Are there resources available to aid in further research of this compound?
A4: While specific research infrastructure dedicated to this compound might not be explicitly mentioned in the provided abstracts, several resources contribute to advancing research on this compound and similar organic molecules. Standard tools like X-ray diffraction [], nuclear magnetic resonance (NMR) spectroscopy [], and infrared (IR) spectroscopy [] offer valuable structural insights. Computational chemistry methods, although not explicitly mentioned in the context of this compound, could be employed to model its properties and reactivity. Access to synthetic methodologies and analytical techniques, coupled with publicly available chemical databases, further empowers researchers to explore the synthesis, characterization, and potential applications of this compound. Continued research on this compound and its derivatives could uncover novel synthetic pathways, unveil unique properties, and potentially lead to applications in diverse fields.
Disclaimer and Information on In-Vitro Research Products
Please be aware that all articles and product information presented on BenchChem are intended solely for informational purposes. The products available for purchase on BenchChem are specifically designed for in-vitro studies, which are conducted outside of living organisms. In-vitro studies, derived from the Latin term "in glass," involve experiments performed in controlled laboratory settings using cells or tissues. It is important to note that these products are not categorized as medicines or drugs, and they have not received approval from the FDA for the prevention, treatment, or cure of any medical condition, ailment, or disease. We must emphasize that any form of bodily introduction of these products into humans or animals is strictly prohibited by law. It is essential to adhere to these guidelines to ensure compliance with legal and ethical standards in research and experimentation.