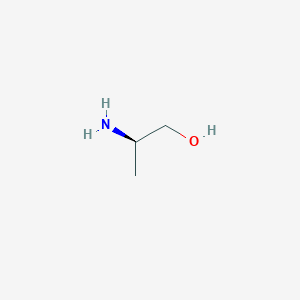
d-Alaninol
Overview
Description
d-Alaninol: is a chiral amino alcohol with the chemical formula C3H9NO . It is a clear, colorless to light yellow liquid that is soluble in water. This compound is classified as an amino alcohol due to the presence of both an amino group (-NH2) and a hydroxyl group (-OH) on adjacent carbon atoms. This compound is an important intermediate in organic synthesis and has broad applications in pharmaceuticals, surfactants, and other chemical industries .
Mechanism of Action
Target of Action
d-Alaninol, also known as ®-(-)-2-Amino-1-propanol, is a chiral secondary amine . It is a biochemical reagent that can be used as a biological material or organic compound for life science related research
Mode of Action
It has been used as a derivatizing agent for gossypol during the analysis of gossypol enantiomers in cottonseed by high-performance liquid chromatography .
Biochemical Pathways
It is known that chiral amino alcohols like this compound are core building units in many drugs, surfactants, and chemicals . For instance, amino propanol is a key intermediate in the synthesis of Ofloxacin, a basic drug in China .
Result of Action
Its role as a biochemical reagent and its use in the synthesis of various compounds suggest that it may have diverse effects depending on the specific context of its use .
Action Environment
It is known that this compound is hygroscopic and should be stored in a dark place under an inert atmosphere .
Biochemical Analysis
Biochemical Properties
d-Alaninol plays a significant role in biochemical reactions. It interacts with various enzymes, proteins, and other biomolecules. For instance, it has been observed to interact with the enzyme Coenzyme B(12)-dependent ethanolamine ammonia-lyase .
Cellular Effects
It is known to influence cell function, including impacts on cell signaling pathways, gene expression, and cellular metabolism .
Molecular Mechanism
At the molecular level, this compound exerts its effects through binding interactions with biomolecules, enzyme inhibition or activation, and changes in gene expression
Temporal Effects in Laboratory Settings
In laboratory settings, the effects of this compound change over time. This includes information on the product’s stability, degradation, and any long-term effects on cellular function observed in in vitro or in vivo studies .
Metabolic Pathways
This compound is involved in several metabolic pathways. It interacts with various enzymes or cofactors, and can affect metabolic flux or metabolite levels .
Preparation Methods
Synthetic Routes and Reaction Conditions: d-Alaninol can be synthesized by the reduction of d-alanine. One common method involves the use of a strong reducing agent such as lithium aluminium hydride (LiAlH4) to convert the carboxylic acid group of d-alanine to an alcohol group . The reaction typically proceeds under anhydrous conditions to prevent the hydrolysis of the reducing agent.
Industrial Production Methods: In industrial settings, this compound is often produced through catalytic hydrogenation of d-alanine using a suitable catalyst such as palladium on carbon (Pd/C). This method is preferred for large-scale production due to its efficiency and cost-effectiveness .
Chemical Reactions Analysis
Types of Reactions: d-Alaninol undergoes various chemical reactions, including:
Oxidation: this compound can be oxidized to form d-alanine or other related compounds.
Reduction: It can be further reduced to form simpler alcohols.
Substitution: The amino group can participate in substitution reactions to form derivatives such as amides or esters.
Common Reagents and Conditions:
Oxidation: Common oxidizing agents include potassium permanganate (KMnO4) and chromium trioxide (CrO3).
Reduction: Lithium aluminium hydride (LiAlH4) is commonly used for reduction reactions.
Substitution: Reagents such as acyl chlorides or anhydrides are used for substitution reactions.
Major Products:
Oxidation: d-Alanine
Reduction: Simpler alcohols
Substitution: Amides, esters, and other derivatives.
Scientific Research Applications
d-Alaninol has a wide range of applications in scientific research:
Chemistry: It is used as a chiral building block in the synthesis of various complex molecules, including pharmaceuticals and agrochemicals.
Biology: this compound is used in the study of enzyme mechanisms and as a substrate in biochemical assays.
Medicine: It serves as an intermediate in the synthesis of drugs such as ofloxacin and levofloxacin, which are important antibiotics.
Industry: this compound is used as an emulsifier and in the production of surfactants
Comparison with Similar Compounds
l-Alaninol: The enantiomer of d-Alaninol, with similar chemical properties but different optical activity.
2-Amino-1-propanol: A related amino alcohol with similar functional groups but different stereochemistry.
Ethanolamine: A simpler amino alcohol with one less carbon atom.
Uniqueness: this compound is unique due to its chiral nature, which makes it valuable in the synthesis of enantiomerically pure compounds. Its specific configuration allows it to be used in the production of chiral drugs and other specialized chemicals .
Properties
IUPAC Name |
(2R)-2-aminopropan-1-ol | |
---|---|---|
Source | PubChem | |
URL | https://pubchem.ncbi.nlm.nih.gov | |
Description | Data deposited in or computed by PubChem | |
InChI |
InChI=1S/C3H9NO/c1-3(4)2-5/h3,5H,2,4H2,1H3/t3-/m1/s1 | |
Source | PubChem | |
URL | https://pubchem.ncbi.nlm.nih.gov | |
Description | Data deposited in or computed by PubChem | |
InChI Key |
BKMMTJMQCTUHRP-GSVOUGTGSA-N | |
Source | PubChem | |
URL | https://pubchem.ncbi.nlm.nih.gov | |
Description | Data deposited in or computed by PubChem | |
Canonical SMILES |
CC(CO)N | |
Source | PubChem | |
URL | https://pubchem.ncbi.nlm.nih.gov | |
Description | Data deposited in or computed by PubChem | |
Isomeric SMILES |
C[C@H](CO)N | |
Source | PubChem | |
URL | https://pubchem.ncbi.nlm.nih.gov | |
Description | Data deposited in or computed by PubChem | |
Molecular Formula |
C3H9NO | |
Source | PubChem | |
URL | https://pubchem.ncbi.nlm.nih.gov | |
Description | Data deposited in or computed by PubChem | |
DSSTOX Substance ID |
DTXSID30904764 | |
Record name | (R)-2-Aminopropanol | |
Source | EPA DSSTox | |
URL | https://comptox.epa.gov/dashboard/DTXSID30904764 | |
Description | DSSTox provides a high quality public chemistry resource for supporting improved predictive toxicology. | |
Molecular Weight |
75.11 g/mol | |
Source | PubChem | |
URL | https://pubchem.ncbi.nlm.nih.gov | |
Description | Data deposited in or computed by PubChem | |
Physical Description |
Clear colorless liquid; [Sigma-Aldrich MSDS] | |
Record name | (R)-(-)-2-Amino-1-propanol | |
Source | Haz-Map, Information on Hazardous Chemicals and Occupational Diseases | |
URL | https://haz-map.com/Agents/10983 | |
Description | Haz-Map® is an occupational health database designed for health and safety professionals and for consumers seeking information about the adverse effects of workplace exposures to chemical and biological agents. | |
Explanation | Copyright (c) 2022 Haz-Map(R). All rights reserved. Unless otherwise indicated, all materials from Haz-Map are copyrighted by Haz-Map(R). No part of these materials, either text or image may be used for any purpose other than for personal use. Therefore, reproduction, modification, storage in a retrieval system or retransmission, in any form or by any means, electronic, mechanical or otherwise, for reasons other than personal use, is strictly prohibited without prior written permission. | |
CAS No. |
35320-23-1 | |
Record name | 2-Aminopropanol, (-)- | |
Source | ChemIDplus | |
URL | https://pubchem.ncbi.nlm.nih.gov/substance/?source=chemidplus&sourceid=0035320231 | |
Description | ChemIDplus is a free, web search system that provides access to the structure and nomenclature authority files used for the identification of chemical substances cited in National Library of Medicine (NLM) databases, including the TOXNET system. | |
Record name | (R)-2-Aminopropanol | |
Source | EPA DSSTox | |
URL | https://comptox.epa.gov/dashboard/DTXSID30904764 | |
Description | DSSTox provides a high quality public chemistry resource for supporting improved predictive toxicology. | |
Record name | (R)-(-)-2-Amino-1-propanol | |
Source | European Chemicals Agency (ECHA) | |
URL | https://echa.europa.eu/information-on-chemicals | |
Description | The European Chemicals Agency (ECHA) is an agency of the European Union which is the driving force among regulatory authorities in implementing the EU's groundbreaking chemicals legislation for the benefit of human health and the environment as well as for innovation and competitiveness. | |
Explanation | Use of the information, documents and data from the ECHA website is subject to the terms and conditions of this Legal Notice, and subject to other binding limitations provided for under applicable law, the information, documents and data made available on the ECHA website may be reproduced, distributed and/or used, totally or in part, for non-commercial purposes provided that ECHA is acknowledged as the source: "Source: European Chemicals Agency, http://echa.europa.eu/". Such acknowledgement must be included in each copy of the material. ECHA permits and encourages organisations and individuals to create links to the ECHA website under the following cumulative conditions: Links can only be made to webpages that provide a link to the Legal Notice page. | |
Record name | 2-AMINOPROPANOL, (-)- | |
Source | FDA Global Substance Registration System (GSRS) | |
URL | https://gsrs.ncats.nih.gov/ginas/app/beta/substances/770ZI70L3Q | |
Description | The FDA Global Substance Registration System (GSRS) enables the efficient and accurate exchange of information on what substances are in regulated products. Instead of relying on names, which vary across regulatory domains, countries, and regions, the GSRS knowledge base makes it possible for substances to be defined by standardized, scientific descriptions. | |
Explanation | Unless otherwise noted, the contents of the FDA website (www.fda.gov), both text and graphics, are not copyrighted. They are in the public domain and may be republished, reprinted and otherwise used freely by anyone without the need to obtain permission from FDA. Credit to the U.S. Food and Drug Administration as the source is appreciated but not required. | |
Retrosynthesis Analysis
AI-Powered Synthesis Planning: Our tool employs the Template_relevance Pistachio, Template_relevance Bkms_metabolic, Template_relevance Pistachio_ringbreaker, Template_relevance Reaxys, Template_relevance Reaxys_biocatalysis model, leveraging a vast database of chemical reactions to predict feasible synthetic routes.
One-Step Synthesis Focus: Specifically designed for one-step synthesis, it provides concise and direct routes for your target compounds, streamlining the synthesis process.
Accurate Predictions: Utilizing the extensive PISTACHIO, BKMS_METABOLIC, PISTACHIO_RINGBREAKER, REAXYS, REAXYS_BIOCATALYSIS database, our tool offers high-accuracy predictions, reflecting the latest in chemical research and data.
Strategy Settings
Precursor scoring | Relevance Heuristic |
---|---|
Min. plausibility | 0.01 |
Model | Template_relevance |
Template Set | Pistachio/Bkms_metabolic/Pistachio_ringbreaker/Reaxys/Reaxys_biocatalysis |
Top-N result to add to graph | 6 |
Feasible Synthetic Routes
Disclaimer and Information on In-Vitro Research Products
Please be aware that all articles and product information presented on BenchChem are intended solely for informational purposes. The products available for purchase on BenchChem are specifically designed for in-vitro studies, which are conducted outside of living organisms. In-vitro studies, derived from the Latin term "in glass," involve experiments performed in controlled laboratory settings using cells or tissues. It is important to note that these products are not categorized as medicines or drugs, and they have not received approval from the FDA for the prevention, treatment, or cure of any medical condition, ailment, or disease. We must emphasize that any form of bodily introduction of these products into humans or animals is strictly prohibited by law. It is essential to adhere to these guidelines to ensure compliance with legal and ethical standards in research and experimentation.