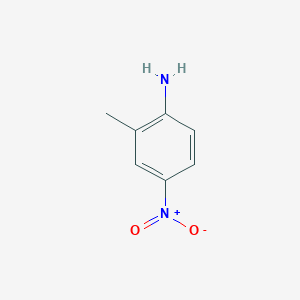
2-Methyl-4-nitroaniline
Overview
Description
2-Methyl-4-nitroaniline is an organic compound with the molecular formula C7H8N2O2. It appears as a yellow to orange crystalline solid and is known for its stability and solubility in organic solvents like ethanol, chloroform, and dimethyl sulfoxide. This compound is primarily used as an intermediate in the synthesis of dyes, pigments, and other organic compounds .
Mechanism of Action
Target of Action
2-Methyl-4-nitroaniline is a versatile compound widely used in various industries . It serves as an intermediate for organic synthesis in the production of dyes, pharmaceuticals, and specialty chemicals . The primary targets of this compound are the biochemical pathways involved in these processes .
Mode of Action
The compound participates in diverse reactions, such as coupling and condensation, enabling the synthesis of new compounds . It also finds application in polymer chemistry as a monomer or building block for producing polymers with desired properties .
Biochemical Pathways
It is known that the compound plays a significant role in organic synthesis, participating in diverse reactions such as coupling, condensation, and nucleophilic substitution .
Pharmacokinetics
The pharmacokinetics of this compound suggest predominant urinary excretion, low tissue distribution, and relatively slow clearance . Following oral administration to rats, the majority of the radioactivity derived from [14C] MNA was excreted in urine, with 75-79% of the dose recovered within 72 hours .
Result of Action
The molecular and cellular effects of this compound’s action are largely dependent on its use. For instance, in the dye industry, this compound acts as a diazo component for synthesizing azo dyes . Its vibrant orange to red colors make it highly desirable for dyeing textiles, leather goods, and paper products .
Action Environment
Environmental factors can influence the action, efficacy, and stability of this compound. For example, the compound is known to be combustible and insoluble in water . Therefore, its storage and handling require specific conditions to prevent heat exposure and moisture . Furthermore, it is classified as toxic and hazardous to the environment, indicating that its use and disposal must be managed carefully to minimize environmental impact .
Biochemical Analysis
Cellular Effects
It has been reported to induce contact hypersensitivity in a human and bacterial mutagenicity . It also influences cell function by affecting the electro-optical properties of organic N-benzyl-2-methyl-4-nitroaniline dispersion in nematic liquid crystals .
Molecular Mechanism
The molecular mechanism of 2-Methyl-4-nitroaniline involves several steps. The first step is N-demethylation, which results in the formation of 4-nitroaniline and formaldehyde . The second step is a monooxygenation, transforming 4-nitroaniline to 4-aminophenol . The third step involves oxidative deamination, leading to the formation of 1,2,4-benzenetriol .
Temporal Effects in Laboratory Settings
In laboratory settings, the effects of this compound can change over time. For instance, it has been used to deposit polycrystalline thin films on Ag, Cu, and Si by conventional and partially ionized beam deposition . Information on the product’s stability, degradation, and long-term effects on cellular function observed in in vitro or in vivo studies is limited.
Metabolic Pathways
The metabolic pathways involving this compound are not well-understood. A related compound, N-Methyl-4-nitroaniline, has been reported to undergo aerobic degradation by Pseudomonas sp. strain MB-P1 .
Preparation Methods
Synthetic Routes and Reaction Conditions: The synthesis of 2-Methyl-4-nitroaniline typically involves the nitration of o-toluidine. The process begins with the protection of the amino group in o-toluidine using acetic anhydride to form an acetamide derivative. This intermediate is then nitrated using concentrated nitric acid to introduce the nitro group at the para position. Finally, the acetamide group is hydrolyzed using sulfuric acid to yield this compound .
Industrial Production Methods: In industrial settings, the production of this compound follows a similar route but on a larger scale. The process involves the use of ortho-toluidine as the starting material, which undergoes acylation, nitration, and hydrolysis. The reaction conditions are optimized to ensure high yield and purity of the final product .
Chemical Reactions Analysis
Types of Reactions: 2-Methyl-4-nitroaniline undergoes various chemical reactions, including:
Reduction: The nitro group can be reduced to an amino group using reducing agents like hydrogen gas in the presence of a catalyst or using chemical reductants like iron and hydrochloric acid.
Substitution: The compound can participate in electrophilic aromatic substitution reactions, where the nitro group directs incoming substituents to the meta position relative to itself.
Common Reagents and Conditions:
Reduction: Hydrogen gas with a palladium catalyst or iron filings with hydrochloric acid.
Substitution: Electrophiles like bromine in the presence of a Lewis acid catalyst.
Oxidation: Potassium permanganate in an acidic medium.
Major Products Formed:
Reduction: 2-Methyl-4-phenylenediamine.
Substitution: 2-Methyl-4-nitro-3-bromoaniline.
Oxidation: 2-Methyl-4-nitrobenzoic acid.
Scientific Research Applications
2-Methyl-4-nitroaniline has several applications in scientific research:
Chemistry: It is used as an intermediate in the synthesis of various dyes and pigments. Its derivatives are used in the production of azo dyes.
Biology: The compound is used in the study of enzyme-catalyzed reactions involving nitroaromatic compounds.
Medicine: It serves as a precursor in the synthesis of pharmaceutical compounds.
Industry: It is used in the production of coatings, plastics, and rubber chemicals.
Comparison with Similar Compounds
2-Methyl-6-nitroaniline: Similar in structure but with the nitro group at the 6-position.
4-Nitro-o-toluidine: Similar in structure but with the nitro group at the 4-position relative to the methyl group.
Uniqueness: 2-Methyl-4-nitroaniline is unique due to its specific substitution pattern, which influences its reactivity and applications. The position of the nitro and methyl groups allows for selective reactions and makes it a valuable intermediate in organic synthesis .
Properties
IUPAC Name |
2-methyl-4-nitroaniline | |
---|---|---|
Source | PubChem | |
URL | https://pubchem.ncbi.nlm.nih.gov | |
Description | Data deposited in or computed by PubChem | |
InChI |
InChI=1S/C7H8N2O2/c1-5-4-6(9(10)11)2-3-7(5)8/h2-4H,8H2,1H3 | |
Source | PubChem | |
URL | https://pubchem.ncbi.nlm.nih.gov | |
Description | Data deposited in or computed by PubChem | |
InChI Key |
XTTIQGSLJBWVIV-UHFFFAOYSA-N | |
Source | PubChem | |
URL | https://pubchem.ncbi.nlm.nih.gov | |
Description | Data deposited in or computed by PubChem | |
Canonical SMILES |
CC1=C(C=CC(=C1)[N+](=O)[O-])N | |
Source | PubChem | |
URL | https://pubchem.ncbi.nlm.nih.gov | |
Description | Data deposited in or computed by PubChem | |
Molecular Formula |
C7H8N2O2 | |
Record name | 2-METHYL-4-NITROANILINE | |
Source | CAMEO Chemicals | |
URL | https://cameochemicals.noaa.gov/chemical/20670 | |
Description | CAMEO Chemicals is a chemical database designed for people who are involved in hazardous material incident response and planning. CAMEO Chemicals contains a library with thousands of datasheets containing response-related information and recommendations for hazardous materials that are commonly transported, used, or stored in the United States. CAMEO Chemicals was developed by the National Oceanic and Atmospheric Administration's Office of Response and Restoration in partnership with the Environmental Protection Agency's Office of Emergency Management. | |
Explanation | CAMEO Chemicals and all other CAMEO products are available at no charge to those organizations and individuals (recipients) responsible for the safe handling of chemicals. However, some of the chemical data itself is subject to the copyright restrictions of the companies or organizations that provided the data. | |
Source | PubChem | |
URL | https://pubchem.ncbi.nlm.nih.gov | |
Description | Data deposited in or computed by PubChem | |
DSSTOX Substance ID |
DTXSID3025629 | |
Record name | 2-Methyl-4-nitroaniline | |
Source | EPA DSSTox | |
URL | https://comptox.epa.gov/dashboard/DTXSID3025629 | |
Description | DSSTox provides a high quality public chemistry resource for supporting improved predictive toxicology. | |
Molecular Weight |
152.15 g/mol | |
Source | PubChem | |
URL | https://pubchem.ncbi.nlm.nih.gov | |
Description | Data deposited in or computed by PubChem | |
Physical Description |
2-methyl-4-nitroaniline appears as yellow needles or mustard yellow powder. (NTP, 1992) | |
Record name | 2-METHYL-4-NITROANILINE | |
Source | CAMEO Chemicals | |
URL | https://cameochemicals.noaa.gov/chemical/20670 | |
Description | CAMEO Chemicals is a chemical database designed for people who are involved in hazardous material incident response and planning. CAMEO Chemicals contains a library with thousands of datasheets containing response-related information and recommendations for hazardous materials that are commonly transported, used, or stored in the United States. CAMEO Chemicals was developed by the National Oceanic and Atmospheric Administration's Office of Response and Restoration in partnership with the Environmental Protection Agency's Office of Emergency Management. | |
Explanation | CAMEO Chemicals and all other CAMEO products are available at no charge to those organizations and individuals (recipients) responsible for the safe handling of chemicals. However, some of the chemical data itself is subject to the copyright restrictions of the companies or organizations that provided the data. | |
Solubility |
less than 1 mg/mL at 72 °F (NTP, 1992) | |
Record name | 2-METHYL-4-NITROANILINE | |
Source | CAMEO Chemicals | |
URL | https://cameochemicals.noaa.gov/chemical/20670 | |
Description | CAMEO Chemicals is a chemical database designed for people who are involved in hazardous material incident response and planning. CAMEO Chemicals contains a library with thousands of datasheets containing response-related information and recommendations for hazardous materials that are commonly transported, used, or stored in the United States. CAMEO Chemicals was developed by the National Oceanic and Atmospheric Administration's Office of Response and Restoration in partnership with the Environmental Protection Agency's Office of Emergency Management. | |
Explanation | CAMEO Chemicals and all other CAMEO products are available at no charge to those organizations and individuals (recipients) responsible for the safe handling of chemicals. However, some of the chemical data itself is subject to the copyright restrictions of the companies or organizations that provided the data. | |
Density |
1.1586 at 284 °F (NTP, 1992) - Denser than water; will sink | |
Record name | 2-METHYL-4-NITROANILINE | |
Source | CAMEO Chemicals | |
URL | https://cameochemicals.noaa.gov/chemical/20670 | |
Description | CAMEO Chemicals is a chemical database designed for people who are involved in hazardous material incident response and planning. CAMEO Chemicals contains a library with thousands of datasheets containing response-related information and recommendations for hazardous materials that are commonly transported, used, or stored in the United States. CAMEO Chemicals was developed by the National Oceanic and Atmospheric Administration's Office of Response and Restoration in partnership with the Environmental Protection Agency's Office of Emergency Management. | |
Explanation | CAMEO Chemicals and all other CAMEO products are available at no charge to those organizations and individuals (recipients) responsible for the safe handling of chemicals. However, some of the chemical data itself is subject to the copyright restrictions of the companies or organizations that provided the data. | |
CAS No. |
99-52-5 | |
Record name | 2-METHYL-4-NITROANILINE | |
Source | CAMEO Chemicals | |
URL | https://cameochemicals.noaa.gov/chemical/20670 | |
Description | CAMEO Chemicals is a chemical database designed for people who are involved in hazardous material incident response and planning. CAMEO Chemicals contains a library with thousands of datasheets containing response-related information and recommendations for hazardous materials that are commonly transported, used, or stored in the United States. CAMEO Chemicals was developed by the National Oceanic and Atmospheric Administration's Office of Response and Restoration in partnership with the Environmental Protection Agency's Office of Emergency Management. | |
Explanation | CAMEO Chemicals and all other CAMEO products are available at no charge to those organizations and individuals (recipients) responsible for the safe handling of chemicals. However, some of the chemical data itself is subject to the copyright restrictions of the companies or organizations that provided the data. | |
Record name | 2-Amino-5-nitrotoluene | |
Source | CAS Common Chemistry | |
URL | https://commonchemistry.cas.org/detail?cas_rn=99-52-5 | |
Description | CAS Common Chemistry is an open community resource for accessing chemical information. Nearly 500,000 chemical substances from CAS REGISTRY cover areas of community interest, including common and frequently regulated chemicals, and those relevant to high school and undergraduate chemistry classes. This chemical information, curated by our expert scientists, is provided in alignment with our mission as a division of the American Chemical Society. | |
Explanation | The data from CAS Common Chemistry is provided under a CC-BY-NC 4.0 license, unless otherwise stated. | |
Record name | 4-Nitro-o-toludine | |
Source | ChemIDplus | |
URL | https://pubchem.ncbi.nlm.nih.gov/substance/?source=chemidplus&sourceid=0000099525 | |
Description | ChemIDplus is a free, web search system that provides access to the structure and nomenclature authority files used for the identification of chemical substances cited in National Library of Medicine (NLM) databases, including the TOXNET system. | |
Record name | 2-Methyl-4-nitroaniline | |
Source | DTP/NCI | |
URL | https://dtp.cancer.gov/dtpstandard/servlet/dwindex?searchtype=NSC&outputformat=html&searchlist=5508 | |
Description | The NCI Development Therapeutics Program (DTP) provides services and resources to the academic and private-sector research communities worldwide to facilitate the discovery and development of new cancer therapeutic agents. | |
Explanation | Unless otherwise indicated, all text within NCI products is free of copyright and may be reused without our permission. Credit the National Cancer Institute as the source. | |
Record name | 2-Methyl-4-nitroaniline | |
Source | DTP/NCI | |
URL | https://dtp.cancer.gov/dtpstandard/servlet/dwindex?searchtype=NSC&outputformat=html&searchlist=2075 | |
Description | The NCI Development Therapeutics Program (DTP) provides services and resources to the academic and private-sector research communities worldwide to facilitate the discovery and development of new cancer therapeutic agents. | |
Explanation | Unless otherwise indicated, all text within NCI products is free of copyright and may be reused without our permission. Credit the National Cancer Institute as the source. | |
Record name | Benzenamine, 2-methyl-4-nitro- | |
Source | EPA Chemicals under the TSCA | |
URL | https://www.epa.gov/chemicals-under-tsca | |
Description | EPA Chemicals under the Toxic Substances Control Act (TSCA) collection contains information on chemicals and their regulations under TSCA, including non-confidential content from the TSCA Chemical Substance Inventory and Chemical Data Reporting. | |
Record name | 2-Methyl-4-nitroaniline | |
Source | EPA DSSTox | |
URL | https://comptox.epa.gov/dashboard/DTXSID3025629 | |
Description | DSSTox provides a high quality public chemistry resource for supporting improved predictive toxicology. | |
Record name | 4-nitro-o-toluidine | |
Source | European Chemicals Agency (ECHA) | |
URL | https://echa.europa.eu/substance-information/-/substanceinfo/100.002.511 | |
Description | The European Chemicals Agency (ECHA) is an agency of the European Union which is the driving force among regulatory authorities in implementing the EU's groundbreaking chemicals legislation for the benefit of human health and the environment as well as for innovation and competitiveness. | |
Explanation | Use of the information, documents and data from the ECHA website is subject to the terms and conditions of this Legal Notice, and subject to other binding limitations provided for under applicable law, the information, documents and data made available on the ECHA website may be reproduced, distributed and/or used, totally or in part, for non-commercial purposes provided that ECHA is acknowledged as the source: "Source: European Chemicals Agency, http://echa.europa.eu/". Such acknowledgement must be included in each copy of the material. ECHA permits and encourages organisations and individuals to create links to the ECHA website under the following cumulative conditions: Links can only be made to webpages that provide a link to the Legal Notice page. | |
Record name | 2-METHYL-4-NITROANILINE | |
Source | FDA Global Substance Registration System (GSRS) | |
URL | https://gsrs.ncats.nih.gov/ginas/app/beta/substances/FFY36EWC4R | |
Description | The FDA Global Substance Registration System (GSRS) enables the efficient and accurate exchange of information on what substances are in regulated products. Instead of relying on names, which vary across regulatory domains, countries, and regions, the GSRS knowledge base makes it possible for substances to be defined by standardized, scientific descriptions. | |
Explanation | Unless otherwise noted, the contents of the FDA website (www.fda.gov), both text and graphics, are not copyrighted. They are in the public domain and may be republished, reprinted and otherwise used freely by anyone without the need to obtain permission from FDA. Credit to the U.S. Food and Drug Administration as the source is appreciated but not required. | |
Melting Point |
268 to 271 °F (NTP, 1992) | |
Record name | 2-METHYL-4-NITROANILINE | |
Source | CAMEO Chemicals | |
URL | https://cameochemicals.noaa.gov/chemical/20670 | |
Description | CAMEO Chemicals is a chemical database designed for people who are involved in hazardous material incident response and planning. CAMEO Chemicals contains a library with thousands of datasheets containing response-related information and recommendations for hazardous materials that are commonly transported, used, or stored in the United States. CAMEO Chemicals was developed by the National Oceanic and Atmospheric Administration's Office of Response and Restoration in partnership with the Environmental Protection Agency's Office of Emergency Management. | |
Explanation | CAMEO Chemicals and all other CAMEO products are available at no charge to those organizations and individuals (recipients) responsible for the safe handling of chemicals. However, some of the chemical data itself is subject to the copyright restrictions of the companies or organizations that provided the data. | |
Synthesis routes and methods
Procedure details
Retrosynthesis Analysis
AI-Powered Synthesis Planning: Our tool employs the Template_relevance Pistachio, Template_relevance Bkms_metabolic, Template_relevance Pistachio_ringbreaker, Template_relevance Reaxys, Template_relevance Reaxys_biocatalysis model, leveraging a vast database of chemical reactions to predict feasible synthetic routes.
One-Step Synthesis Focus: Specifically designed for one-step synthesis, it provides concise and direct routes for your target compounds, streamlining the synthesis process.
Accurate Predictions: Utilizing the extensive PISTACHIO, BKMS_METABOLIC, PISTACHIO_RINGBREAKER, REAXYS, REAXYS_BIOCATALYSIS database, our tool offers high-accuracy predictions, reflecting the latest in chemical research and data.
Strategy Settings
Precursor scoring | Relevance Heuristic |
---|---|
Min. plausibility | 0.01 |
Model | Template_relevance |
Template Set | Pistachio/Bkms_metabolic/Pistachio_ringbreaker/Reaxys/Reaxys_biocatalysis |
Top-N result to add to graph | 6 |
Feasible Synthetic Routes
Q1: What is the molecular formula and weight of 2-Methyl-4-nitroaniline?
A1: this compound has the molecular formula C7H8N2O2 and a molecular weight of 152.15 g/mol. []
Q2: What spectroscopic data is available for MNA?
A2: Extensive spectroscopic characterization of MNA includes Fourier-transform infrared (FT-IR) [, , , , ], Raman [, , ], UV-Vis [, ], and nuclear magnetic resonance (NMR) [] spectroscopy. These studies provide insights into its vibrational modes, electronic transitions, and molecular dynamics.
Q3: Why is MNA considered a promising material for nonlinear optics?
A3: MNA possesses a large second-order nonlinear optical susceptibility (χ(2)), which arises from its asymmetric electronic structure characterized by a donor-acceptor conjugated system. [, , , , ]
Q4: How does the second-harmonic generation (SHG) efficiency of MNA compare to other materials?
A4: MNA exhibits strong SHG efficiency, exceeding that of potassium dihydrogen phosphate (KDP) and comparable to some commercially available NLO crystals. [, ] Its N-benzyl derivative, N-benzyl-2-methyl-4-nitroaniline (BNA), shows even higher SHG efficiency, with a d33 coefficient of 234 pm/V, the largest reported for yellow-colored NLO materials. [, , ]
Q5: What applications exploit the NLO properties of MNA?
A5: The strong SHG efficiency of MNA and its derivatives makes them suitable for various applications, including terahertz (THz) wave generation, optical frequency conversion, and electro-optic modulation. [, , , , , , , , ]
Q6: What methods are used to grow MNA crystals?
A6: MNA crystals can be grown using various techniques, including slow evaporation from solution, Bridgman-Stockbarger method, and physical vapor deposition. [, , , , , , ]
Q7: How does the choice of solvent influence the growth and properties of MNA crystals?
A7: Solvent polarity significantly affects the morphology, growth rate, and optical properties of BNA crystals. Polar aprotic solvents have been shown to promote faster growth and result in crystals with higher THz generation efficiency. [, ]
Q8: Can MNA be deposited as thin films?
A8: Yes, MNA thin films can be fabricated using techniques like ionized cluster beam deposition and vapor deposition. [, ] Controlling the substrate temperature during deposition allows for manipulating the molecular orientation within the film, thereby influencing its NLO properties.
Q9: What computational methods are employed to study the electronic structure and optical properties of MNA?
A9: Density functional theory (DFT) calculations are commonly used to investigate the electronic structure, linear and nonlinear optical properties, and vibrational frequencies of MNA. [, , ] Time-dependent DFT (TDDFT) methods provide insights into its excited-state properties and electronic transitions. []
Q10: How do intermolecular interactions affect the NLO properties of MNA?
A10: Computational studies using the supermolecule approach reveal that intermolecular interactions, such as π-stacking and hydrogen bonding, influence the hyperpolarizability of MNA. [, ] These interactions contribute to the overall macroscopic NLO response of the crystal.
Q11: How do structural modifications affect the NLO properties of MNA?
A11: Introducing substituents on the amino group of MNA, such as alkyl groups or benzyl groups, can significantly impact its NLO properties. [, , ] For instance, N-benzyl-MNA (BNA) exhibits enhanced SHG efficiency compared to the parent MNA molecule. [, ]
Q12: What is the impact of deuteration on the properties of MNA?
A12: Studies on deuterated MNA crystals reveal that deuteration influences the molecular dynamics and activation energies for reorientation of various functional groups. [] This, in turn, can affect its NLO properties and thermal stability.
Disclaimer and Information on In-Vitro Research Products
Please be aware that all articles and product information presented on BenchChem are intended solely for informational purposes. The products available for purchase on BenchChem are specifically designed for in-vitro studies, which are conducted outside of living organisms. In-vitro studies, derived from the Latin term "in glass," involve experiments performed in controlled laboratory settings using cells or tissues. It is important to note that these products are not categorized as medicines or drugs, and they have not received approval from the FDA for the prevention, treatment, or cure of any medical condition, ailment, or disease. We must emphasize that any form of bodily introduction of these products into humans or animals is strictly prohibited by law. It is essential to adhere to these guidelines to ensure compliance with legal and ethical standards in research and experimentation.