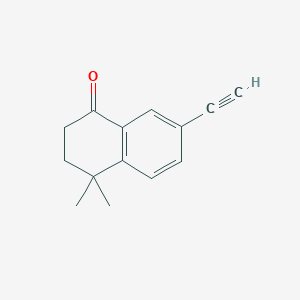
4,4-Dimethyl-7-ethynyl-1-tetralone
Overview
Description
4,4-Dimethyl-7-ethynyl-1-tetralone is a chemical compound with the molecular formula C14H14O and a molecular weight of 198.26 g/mol . It is primarily used as an intermediate in the production of high-affinity retinoic acid receptor antagonists . This compound is notable for its unique structure, which includes a tetralone core with ethynyl and dimethyl substituents.
Mechanism of Action
Target of Action
The primary target of 4,4-Dimethyl-7-ethynyl-1-tetralone is the retinoic acid receptor (RAR) . RARs are a class of nuclear receptors that regulate gene expression, cellular growth, and differentiation. They play a crucial role in the development, maintenance, and function of various tissues in the body .
Mode of Action
This compound interacts with RARs as an antagonist . As an antagonist, it binds to the receptor and inhibits its activation, thereby preventing the receptor’s normal function. This interaction leads to changes in gene expression and cellular processes regulated by RARs .
Biochemical Pathways
The compound’s interaction with RARs affects the retinoic acid signaling pathway . This pathway is involved in various biological processes, including cell proliferation, differentiation, and apoptosis. By acting as an antagonist, this compound can modulate these processes and influence the downstream effects of the pathway .
Result of Action
The molecular and cellular effects of this compound’s action are primarily related to its antagonistic activity on RARs . By inhibiting the activation of these receptors, the compound can modulate gene expression and cellular processes regulated by the retinoic acid signaling pathway . This modulation can have various effects, depending on the specific cellular context .
Action Environment
Environmental factors can influence the action, efficacy, and stability of this compound. Factors such as pH, temperature, and the presence of other molecules can affect the compound’s stability and its interaction with RARs . Additionally, the compound’s efficacy can be influenced by factors such as the concentration of RARs and the presence of other signaling molecules .
Biochemical Analysis
Biochemical Properties
It is known to be an intermediate in the production of high-affinity retinoic acid receptor (RAR) antagonists . This suggests that it may interact with RARs and other associated biomolecules in biochemical reactions.
Cellular Effects
Given its role as an intermediate in the production of RAR antagonists , it may influence cell function by modulating retinoic acid signaling pathways.
Molecular Mechanism
As an intermediate in the production of RAR antagonists , it may exert its effects at the molecular level by binding to RARs, inhibiting their activity, and causing changes in gene expression.
Preparation Methods
The synthesis of 4,4-Dimethyl-7-ethynyl-1-tetralone typically involves several steps, starting from readily available precursors. One common synthetic route involves the alkylation of a tetralone derivative followed by ethynylation. The reaction conditions often require the use of strong bases and specific catalysts to achieve high yields . Industrial production methods may involve similar steps but are optimized for large-scale synthesis, ensuring cost-effectiveness and efficiency.
Chemical Reactions Analysis
4,4-Dimethyl-7-ethynyl-1-tetralone undergoes various chemical reactions, including:
Oxidation: This reaction can convert the tetralone to its corresponding carboxylic acid or other oxidized forms.
Reduction: Reduction reactions can yield alcohols or other reduced derivatives.
Substitution: The ethynyl group can be substituted with other functional groups under appropriate conditions. Common reagents used in these reactions include oxidizing agents like potassium permanganate, reducing agents like lithium aluminum hydride, and various nucleophiles for substitution reactions. The major products formed depend on the specific reaction conditions and reagents used.
Scientific Research Applications
4,4-Dimethyl-7-ethynyl-1-tetralone has several scientific research applications:
Chemistry: It is used as an intermediate in the synthesis of complex organic molecules.
Biology: The compound is studied for its interactions with biological macromolecules.
Medicine: It serves as a precursor in the development of pharmaceuticals, particularly retinoic acid receptor antagonists.
Industry: The compound is utilized in the production of various chemical products and materials.
Comparison with Similar Compounds
4,4-Dimethyl-7-ethynyl-1-tetralone can be compared with other similar compounds, such as:
4-Hydroxy-4,7-dimethyl-1-tetralone: This compound has a hydroxyl group instead of an ethynyl group, leading to different chemical properties and reactivity.
4,4-Dimethyl-1-tetralone: Lacks the ethynyl group, which significantly alters its chemical behavior and applications. The uniqueness of this compound lies in its specific substituents, which confer distinct chemical and biological properties.
Properties
IUPAC Name |
7-ethynyl-4,4-dimethyl-2,3-dihydronaphthalen-1-one | |
---|---|---|
Source | PubChem | |
URL | https://pubchem.ncbi.nlm.nih.gov | |
Description | Data deposited in or computed by PubChem | |
InChI |
InChI=1S/C14H14O/c1-4-10-5-6-12-11(9-10)13(15)7-8-14(12,2)3/h1,5-6,9H,7-8H2,2-3H3 | |
Source | PubChem | |
URL | https://pubchem.ncbi.nlm.nih.gov | |
Description | Data deposited in or computed by PubChem | |
InChI Key |
FSENXSHCDBCKEZ-UHFFFAOYSA-N | |
Source | PubChem | |
URL | https://pubchem.ncbi.nlm.nih.gov | |
Description | Data deposited in or computed by PubChem | |
Canonical SMILES |
CC1(CCC(=O)C2=C1C=CC(=C2)C#C)C | |
Source | PubChem | |
URL | https://pubchem.ncbi.nlm.nih.gov | |
Description | Data deposited in or computed by PubChem | |
Molecular Formula |
C14H14O | |
Source | PubChem | |
URL | https://pubchem.ncbi.nlm.nih.gov | |
Description | Data deposited in or computed by PubChem | |
DSSTOX Substance ID |
DTXSID50444339 | |
Record name | 4,4-DIMETHYL-7-ETHYNYL-1-TETRALONE | |
Source | EPA DSSTox | |
URL | https://comptox.epa.gov/dashboard/DTXSID50444339 | |
Description | DSSTox provides a high quality public chemistry resource for supporting improved predictive toxicology. | |
Molecular Weight |
198.26 g/mol | |
Source | PubChem | |
URL | https://pubchem.ncbi.nlm.nih.gov | |
Description | Data deposited in or computed by PubChem | |
CAS No. |
166978-48-9 | |
Record name | 4,4-DIMETHYL-7-ETHYNYL-1-TETRALONE | |
Source | EPA DSSTox | |
URL | https://comptox.epa.gov/dashboard/DTXSID50444339 | |
Description | DSSTox provides a high quality public chemistry resource for supporting improved predictive toxicology. | |
Synthesis routes and methods
Procedure details
Disclaimer and Information on In-Vitro Research Products
Please be aware that all articles and product information presented on BenchChem are intended solely for informational purposes. The products available for purchase on BenchChem are specifically designed for in-vitro studies, which are conducted outside of living organisms. In-vitro studies, derived from the Latin term "in glass," involve experiments performed in controlled laboratory settings using cells or tissues. It is important to note that these products are not categorized as medicines or drugs, and they have not received approval from the FDA for the prevention, treatment, or cure of any medical condition, ailment, or disease. We must emphasize that any form of bodily introduction of these products into humans or animals is strictly prohibited by law. It is essential to adhere to these guidelines to ensure compliance with legal and ethical standards in research and experimentation.