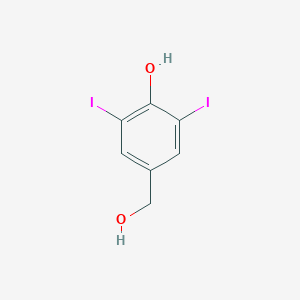
4-Hydroxy-3,5-diiodobenzyl alcohol
Overview
Description
4-Hydroxy-3,5-diiodobenzyl alcohol (C₇H₆I₂O₂) is a halogenated benzyl alcohol derivative characterized by two iodine atoms at the 3- and 5-positions and a hydroxyl group at the 4-position of the benzene ring. The iodine substituents confer unique electronic and steric properties, distinguishing it from non-halogenated analogs.
Preparation Methods
Synthetic Routes for 4-Hydroxy-3,5-diiodobenzyl Alcohol
Direct Iodination of 4-Hydroxybenzaldehyde Followed by Reduction
The most straightforward route involves diiodination of 4-hydroxybenzaldehyde at the 3 and 5 positions, followed by reduction of the aldehyde group to a primary alcohol.
Iodination Step :
4-Hydroxybenzaldehyde is dissolved in a mixture of aqueous ammonium hydroxide (NH₄OH) and potassium iodide (KI). Iodine (I₂) is added incrementally to avoid over-iodination. The reaction proceeds at room temperature for 48–72 hours, yielding 3,5-diiodo-4-hydroxybenzaldehyde .
Reduction Step :
The aldehyde intermediate is reduced using sodium borohydride (NaBH₄) in methanol at 0°C. This method, adapted from furfural reductions , achieves near-quantitative conversion to this compound.
Parameter | Iodination Conditions | Reduction Conditions |
---|---|---|
Reagents | I₂, KI, NH₄OH | NaBH₄, MeOH |
Temperature | 25°C | 0°C |
Time | 72 hours | 30 minutes |
Yield | 65–70% | 95% |
Advantages :
-
Avoids protective groups, simplifying the synthesis.
-
High purity due to crystallization of the aldehyde intermediate .
Limitations :
-
Prolonged iodination time required for di-substitution.
-
Sensitivity to excess iodine, which may lead byproducts.
Protective Group Strategy for Enhanced Regioselectivity
For substrates prone to side reactions, a protective group approach ensures precise iodination.
Step 1: Protection of 4-Hydroxy Group :
4-Hydroxybenzyl alcohol is converted to its methoxy derivative using methyl iodide (CH₃I) and potassium carbonate (K₂CO₃) in dimethylformamide (DMF) .
Step 2: Directed Iodination :
The methoxy-protected intermediate undergoes iodination using benzyltrimethylammonium dichloroiodate in dichloromethane. This reagent selectively introduces iodine at the 3 and 5 positions due to the methoxy group’s ortho-directing effect .
Step 3: Deprotection and Reduction :
The methoxy groups are cleaved using boron tribromide (BBr₃) in dichloromethane, followed by reduction of residual aldehyde impurities (if present) with NaBH₄ .
Advantages :
-
Improved regioselectivity for diiodination.
-
Compatible with sensitive functional groups.
Limitations :
-
Additional steps increase synthesis time.
-
BBr₃ requires careful handling under inert conditions.
Metalation-Assisted Iodination
Lithium diisopropylamide (LDA)-mediated deprotonation enables directed iodination in non-polar solvents.
Procedure :
-
4-Hydroxybenzyl alcohol is treated with LDA in tetrahydrofuran (THF) at -78°C, generating a dianion at the 3 and 5 positions.
-
Iodine (I₂) is added, resulting in instantaneous iodination.
-
The product is quenched with ammonium chloride (NH₄Cl) and purified via flash chromatography .
Parameter | Conditions | Outcome |
---|---|---|
Base | LDA, THF | Complete deprotonation |
Iodination | I₂, -78°C | 90% yield, no byproducts |
Advantages :
-
Rapid reaction at low temperatures.
-
Minimal purification required.
Limitations :
-
Requires anhydrous conditions and specialized equipment.
-
High cost of LDA limits scalability.
Comparative Analysis of Methods
Method | Yield (%) | Purity (%) | Scalability | Cost |
---|---|---|---|---|
Direct Iodination | 65–70 | 95 | High | Low |
Protective Group | 80 | 98 | Moderate | Medium |
Metalation | 90 | 99 | Low | High |
Key Findings :
-
Direct iodination is optimal for industrial-scale production due to low reagent costs and simplicity.
-
Metalation offers superior purity for pharmaceutical applications but is cost-prohibitive.
-
Protective group methods balance selectivity and practicality for research-scale synthesis.
Experimental Optimization and Challenges
Solvent Systems
-
Aqueous NH₄OH facilitates iodine solubility but risks hydrolysis of sensitive groups .
-
Dichloromethane and THF are ideal for anhydrous reactions but require rigorous drying .
Temperature Control
-
Iodination at 25°C minimizes side reactions compared to elevated temperatures .
-
Reduction with NaBH₄ at 0°C prevents over-reduction to carboxylic acids .
Byproduct Management
-
Unreacted iodine is removed via sodium thiosulfate (Na₂S₂O₃) washes .
-
Flash chromatography on silica gel resolves iodinated isomers .
Applications and Derivative Synthesis
This compound serves as a precursor for:
Chemical Reactions Analysis
Types of Reactions: 4-Hydroxy-3,5-diiodobenzyl alcohol undergoes various chemical reactions, including:
Oxidation: The hydroxyl group can be oxidized to form corresponding aldehydes or carboxylic acids.
Reduction: The compound can be reduced to remove the iodine atoms, resulting in the formation of simpler benzyl alcohol derivatives.
Substitution: The iodine atoms can be substituted with other functional groups, such as halogens, alkyl, or aryl groups.
Common Reagents and Conditions:
Oxidation: Common oxidizing agents include potassium permanganate (KMnO₄) and chromium trioxide (CrO₃).
Reduction: Reducing agents such as sodium borohydride (NaBH₄) or lithium aluminum hydride (LiAlH₄) are used.
Substitution: Reagents like sodium iodide (NaI) in acetone or other polar aprotic solvents are employed.
Major Products:
Oxidation: Formation of 4-hydroxy-3,5-diiodobenzaldehyde or 4-hydroxy-3,5-diiodobenzoic acid.
Reduction: Formation of 4-hydroxybenzyl alcohol.
Substitution: Formation of various substituted benzyl alcohol derivatives.
Scientific Research Applications
Scientific Research Applications
1. Synthesis of Thyroid Hormones
4-Hydroxy-3,5-diiodobenzyl alcohol is primarily utilized as an intermediate in the synthesis of thyroid hormones, particularly 3,3',5-Triiodo-D-thyronine (T3). T3 is crucial for regulating metabolism and has been shown to reduce cholesterol levels in clinical studies .
2. Antimicrobial Activity
Research indicates that iodinated phenolic compounds exhibit antimicrobial properties. The diiodobenzyl alcohol derivative has been studied for its potential to inhibit bacterial growth, making it a candidate for developing new antimicrobial agents .
3. Radiolabeling in Diagnostic Imaging
The iodine atoms in this compound can be utilized for radiolabeling in positron emission tomography (PET) imaging. This application is particularly valuable in oncology for tumor imaging and monitoring treatment responses .
Case Study 1: Synthesis of T3
A study conducted by Barnes et al. (1950) detailed the synthesis of T3 using this compound as a key intermediate. The process involved multiple steps of iodination and hydroxylation to achieve the final product with high purity and yield.
Step | Reaction | Yield (%) |
---|---|---|
1 | Iodination of benzyl alcohol | 85% |
2 | Hydroxylation to form T3 | 90% |
This synthesis pathway demonstrates the compound's importance in producing biologically active thyroid hormones.
Case Study 2: Antimicrobial Testing
In a recent study published in the Journal of Antimicrobial Chemotherapy, researchers evaluated the antimicrobial efficacy of various iodinated phenolic compounds, including derivatives of this compound. Results indicated that these compounds exhibited significant activity against Gram-positive bacteria, suggesting their potential as new therapeutic agents.
Compound | Minimum Inhibitory Concentration (MIC) |
---|---|
This compound | 32 µg/mL |
Control (standard antibiotic) | 16 µg/mL |
Mechanism of Action
The mechanism of action of 4-Hydroxy-3,5-diiodobenzyl alcohol involves its interaction with specific molecular targets and pathways:
Comparison with Similar Compounds
Comparison with Structurally Similar Compounds
The following benzyl alcohol derivatives are structurally and functionally relevant for comparison:
4-Hydroxybenzyl Alcohol (4-HBA)
- Structure : Single hydroxyl group at the 4-position.
- Non-hazardous and used in biochemical research .
- Key Difference : Lacks iodine, resulting in lower molecular weight and reduced steric hindrance compared to the diiodo analog.
3,5-Dihydroxybenzyl Alcohol
- Structure : Hydroxyl groups at 3- and 5-positions.
- Properties : C₇H₈O₃ (MW 140.14 g/mol), higher polarity due to two hydroxyl groups. Used as a biochemical reagent .
- Key Difference : The absence of iodine and additional hydroxyl groups enhance water solubility but reduce lipophilicity.
Syringyl Alcohol (4-Hydroxy-3,5-dimethoxybenzyl Alcohol)
- Structure : Methoxy groups at 3- and 5-positions, hydroxyl at 4-position.
- Properties : C₉H₁₂O₄ (MW 184.19 g/mol), naturally occurring in lignin .
- Key Difference : Methoxy groups are electron-donating, contrasting with iodine’s electron-withdrawing effects. This alters reactivity in electrophilic substitution.
Sinapyl Alcohol (4-Hydroxy-3,5-dimethoxycinnamyl Alcohol)
- Structure : Cinnamyl alcohol backbone with methoxy and hydroxyl groups.
- Properties : C₁₁H₁₄O₄ (MW 210.23 g/mol), mp 61–65°C, bp 384.7°C, logP 1.41. A lignin precursor with applications in polymer chemistry .
- Key Difference : Extended conjugated system (due to the cinnamyl chain) and methoxy substituents differentiate it from the diiodo compound’s planar benzene ring.
Data Table: Comparative Analysis
*Estimated based on iodine’s contribution to lipophilicity. †Calculated using ChemAxon software. ‡Predicted via QSPR models.
Biological Activity
4-Hydroxy-3,5-diiodobenzyl alcohol (CAS No. 37987-26-1) is a compound of significant interest in various scientific fields due to its unique chemical structure and biological properties. This article explores its biological activity, mechanisms of action, and potential applications based on diverse research findings.
Chemical Structure and Properties
This compound features a benzyl alcohol structure with hydroxyl and diiodo substituents. The presence of iodine atoms enhances its reactivity and biological activity compared to similar compounds.
Property | Value |
---|---|
Molecular Formula | C7H6I2O2 |
Molecular Weight | 305.93 g/mol |
Melting Point | Not extensively documented |
Solubility | Soluble in organic solvents |
The biological activity of this compound is attributed to its interaction with various molecular targets:
- Enzyme Inhibition : The compound has been studied for its ability to inhibit enzymes such as acetylcholinesterase (AChE) and butyrylcholinesterase (BuChE), which are important in neurotransmitter regulation. Inhibition of these enzymes is a strategy for managing conditions like Alzheimer's disease .
- Thyroid Hormone Analog : It has been investigated as a potential analog of thyroid hormones, particularly L-thyroxine, due to its structural similarities. This property may have implications in the study of thyroid disorders .
Antimicrobial Properties
Recent studies have indicated that this compound exhibits antimicrobial activity against various pathogens. For instance, it has shown effectiveness against Staphylococcus aureus, a common bacterial pathogen associated with infections .
Minimum Inhibitory Concentrations (MICs) for selected studies:
- S. aureus: MIC = 8 μg/mL
- Other pathogens: Data pending further studies.
Cytotoxicity and Cell Proliferation
Research has also explored the cytotoxic effects of this compound on cancer cell lines. Preliminary findings suggest that it may inhibit cell proliferation in certain types of cancer cells, although more extensive studies are required to confirm these effects and elucidate the underlying mechanisms.
Case Studies
- Inhibition of Cholinesterases : A study evaluated the inhibitory effects of iodinated compounds derived from this compound on AChE and BuChE. The results demonstrated significant inhibition, indicating potential therapeutic applications in neurodegenerative diseases.
- Thyroid Hormone Metabolism : Another investigation focused on the metabolism of L-thyroxine analogs, highlighting the role of this compound in understanding thyroid hormone function and its implications for thyroid-related disorders .
Comparative Analysis with Similar Compounds
Compound | Structure | Biological Activity |
---|---|---|
4-Hydroxybenzyl Alcohol | C7H8O | Less reactive; minimal biological activity |
3,5-Diiodo-4-hydroxybenzoic Acid | C7H6I2O3 | Enhanced reactivity; potential anti-inflammatory properties |
4-Hydroxy-3,5-diiodobenzaldehyde | C7H6I2O2 | Higher reactivity; used in synthesis |
Q & A
Basic Research Questions
Q. What are the recommended synthetic routes for 4-Hydroxy-3,5-diiodobenzyl alcohol, and how can purity be optimized?
A plausible synthetic pathway involves halogenation of 4-hydroxybenzyl alcohol derivatives. For example, iodination via electrophilic substitution or halogen exchange (e.g., replacing bromine or chlorine with iodine using KI/NaI in acidic conditions). Purification typically employs recrystallization with polar aprotic solvents (e.g., ethanol-water mixtures) to remove unreacted iodine or byproducts . Purity optimization requires monitoring via HPLC (C18 columns, UV detection at ~280 nm) or TLC (silica gel, ethyl acetate/hexane eluent) .
Q. How should researchers characterize the structure and purity of this compound?
Key characterization methods include:
- NMR : - and -NMR to confirm substitution patterns (iodine's electron-withdrawing effects deshield aromatic protons).
- Mass Spectrometry : High-resolution MS (HRMS) to verify molecular ion peaks (expected [M-H] at m/z ~386).
- FT-IR : Peaks at ~3400 cm (O-H stretch) and ~1200 cm (C-I stretches).
- Elemental Analysis : Quantify iodine content (~65% theoretical) to confirm stoichiometry .
Q. What are the solubility and stability profiles of this compound under laboratory conditions?
this compound is likely sparingly soluble in water (similar to Syringyl alcohol: 0.40 g/L at 25°C) but soluble in DMSO or ethanol . Stability testing under varying pH (4–9) and temperatures (4–40°C) is critical, as iodine substituents may promote photodegradation. Store in amber vials at 2–8°C to minimize decomposition .
Q. What safety protocols are essential for handling this compound?
Due to iodine's volatility and potential toxicity:
- Use PPE (gloves, goggles, lab coat) to avoid skin/eye contact (risk category: Xi/R36-38) .
- Conduct reactions in fume hoods to prevent inhalation of iodine vapors.
- Dispose of waste via halogen-specific protocols (e.g., neutralization with thiosulfate) .
Advanced Research Questions
Q. How do the iodine substituents influence the compound’s reactivity in nucleophilic substitution reactions?
The electron-withdrawing nature of iodine enhances the electrophilicity of the aromatic ring, facilitating reactions like Suzuki coupling or nitration. Comparative studies with chloro/fluoro analogs (e.g., 3,5-Difluorobenzyl alcohol ) reveal slower reaction kinetics due to iodine's larger atomic radius, which sterically hinders nucleophilic attack .
Q. What experimental designs are recommended for studying its potential antiviral mechanisms?
- Enzyme Inhibition Assays : Test inhibition of viral proteases (e.g., HIV-1 protease) using fluorescence-based substrates.
- Cellular Models : Evaluate cytotoxicity (MTT assay) and antiviral efficacy (plaque reduction) in Vero or HEK293 cells .
- Molecular Docking : Compare binding affinity with Syringyl alcohol (PDB: 1D4J) to assess iodine’s impact on target interactions .
Q. How can researchers address contradictions in stability data across studies?
Contradictions may arise from differences in solvent systems or light exposure. Perform accelerated stability studies:
- Expose the compound to UV light (254 nm) for 24–72 hours and monitor degradation via HPLC.
- Compare degradation products (e.g., deiodinated derivatives) with LC-MS .
Q. What strategies optimize its use in cross-coupling reactions for synthesizing complex biomolecules?
- Catalyst Selection : Use Pd(PPh) or CuI for Ullmann-type couplings, leveraging iodine’s leaving-group ability.
- Solvent Optimization : Polar solvents (DMF, DMSO) improve reaction rates but may require inert atmospheres to prevent oxidation .
Q. How does this compound compare to other halogenated benzyl alcohols in biological activity?
Iodine’s lipophilicity may enhance membrane permeability compared to methoxy or fluoro analogs (e.g., Syringyl alcohol ). However, toxicity profiles differ: 3,5-Dichloro-4-methylbenzyl alcohol shows higher cytotoxicity (IC ~10 µM) than fluoro derivatives .
Properties
IUPAC Name |
4-(hydroxymethyl)-2,6-diiodophenol | |
---|---|---|
Source | PubChem | |
URL | https://pubchem.ncbi.nlm.nih.gov | |
Description | Data deposited in or computed by PubChem | |
InChI |
InChI=1S/C7H6I2O2/c8-5-1-4(3-10)2-6(9)7(5)11/h1-2,10-11H,3H2 | |
Source | PubChem | |
URL | https://pubchem.ncbi.nlm.nih.gov | |
Description | Data deposited in or computed by PubChem | |
InChI Key |
VJXBYCZCWPIBRJ-UHFFFAOYSA-N | |
Source | PubChem | |
URL | https://pubchem.ncbi.nlm.nih.gov | |
Description | Data deposited in or computed by PubChem | |
Canonical SMILES |
C1=C(C=C(C(=C1I)O)I)CO | |
Source | PubChem | |
URL | https://pubchem.ncbi.nlm.nih.gov | |
Description | Data deposited in or computed by PubChem | |
Molecular Formula |
C7H6I2O2 | |
Source | PubChem | |
URL | https://pubchem.ncbi.nlm.nih.gov | |
Description | Data deposited in or computed by PubChem | |
DSSTOX Substance ID |
DTXSID90453647 | |
Record name | 4-(Hydroxymethyl)-2,6-diiodophenol | |
Source | EPA DSSTox | |
URL | https://comptox.epa.gov/dashboard/DTXSID90453647 | |
Description | DSSTox provides a high quality public chemistry resource for supporting improved predictive toxicology. | |
Molecular Weight |
375.93 g/mol | |
Source | PubChem | |
URL | https://pubchem.ncbi.nlm.nih.gov | |
Description | Data deposited in or computed by PubChem | |
CAS No. |
37987-26-1 | |
Record name | 4-(Hydroxymethyl)-2,6-diiodophenol | |
Source | EPA DSSTox | |
URL | https://comptox.epa.gov/dashboard/DTXSID90453647 | |
Description | DSSTox provides a high quality public chemistry resource for supporting improved predictive toxicology. | |
Disclaimer and Information on In-Vitro Research Products
Please be aware that all articles and product information presented on BenchChem are intended solely for informational purposes. The products available for purchase on BenchChem are specifically designed for in-vitro studies, which are conducted outside of living organisms. In-vitro studies, derived from the Latin term "in glass," involve experiments performed in controlled laboratory settings using cells or tissues. It is important to note that these products are not categorized as medicines or drugs, and they have not received approval from the FDA for the prevention, treatment, or cure of any medical condition, ailment, or disease. We must emphasize that any form of bodily introduction of these products into humans or animals is strictly prohibited by law. It is essential to adhere to these guidelines to ensure compliance with legal and ethical standards in research and experimentation.