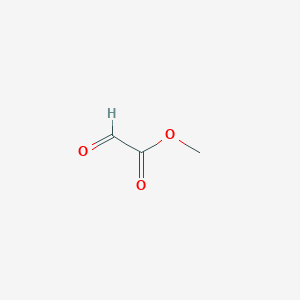
Methyl glyoxylate
Overview
Description
Methyl glyoxylate is an organic compound with the molecular formula C3H4O3. It is an ester derivative of glyoxylic acid, possessing both aldehyde and carboxyl functional groups. This compound is widely used in organic synthesis and chemical production due to its reactivity and versatility.
Mechanism of Action
Target of Action
Methyl glyoxylate, also known as methyl 2-oxoacetate, primarily targets the glyoxalase system . This system comprises of GLYI and GLYII enzymes , and it has emerged as one of the primary methylglyoxal (MG) detoxification pathways with an indispensable role during abiotic and biotic stresses .
Mode of Action
This compound interacts with its targets through a process of enzymatic oxidation . The cascade catalysis of glycolate oxidase from Spinacia oleracea (So GOX), catalase from Helicobacter pylori (Hp CAT), and hemoglobin from Vitreoscilla stercoraria (Vs HGB) was constructed to enable efficient oxidation of methyl glycolate to this compound .
Biochemical Pathways
This compound is involved in the glyoxalase pathway and the glyoxylate cycle . The glyoxalase pathway is responsible for the detoxification of methylglyoxal, a reactive carbonyl species . The glyoxylate cycle, on the other hand, is an anabolic pathway that centers on the conversion of acetyl-CoA to succinate for the synthesis of carbohydrates .
Pharmacokinetics
The pharmacokinetics of this compound involve its transformation through enzymatic oxidation . The use of crude enzyme virtually eliminated side reactions and simplified the preparation of biocatalysts . Under optimized conditions, the crude enzyme Vs HGB-GSG- So GOX mut -GGGGS- Hp CAT catalyzed the oxidation of 200 mM methyl glycolate for 6 h, giving a yield of 95.3% .
Result of Action
The result of this compound’s action is the production of glyoxylic acid . Glyoxylic acid, possessing aldehyde and carboxyl groups, serves as platform chemicals for the synthesis of vanillin, ®-pantolactone, antibiotics, or agrochemicals .
Action Environment
The action of this compound is influenced by environmental factors. For instance, the dynamic responsiveness of the MG-metabolizing GLY pathway to both endogenous cues such as phytohormones, nutrient status, etc., as well as external environmental fluctuations (abiotic and biotic stresses) indicates that a tight regulation occurs in the cell to maintain physiological levels of MG in the system .
Biochemical Analysis
Biochemical Properties
Methyl glyoxylate participates in the glyoxylate cycle, an important pathway involved in the synthesis of carbohydrates from fatty acids or two-carbon precursors . It interacts with enzymes such as isocitrate lyase and malate synthase, which are key enzymes in the glyoxylate cycle . The nature of these interactions involves the conversion of isocitrate to succinate and glyoxylate .
Cellular Effects
This compound has significant effects on various types of cells and cellular processes. It is involved in the regulation of cell function, impacting cell signaling pathways, gene expression, and cellular metabolism . For instance, this compound can covalently modify DNA, RNA, and protein, forming advanced glycation end products (MG-AGEs), which are associated with the onset and progression of many pathologies .
Molecular Mechanism
The molecular mechanism of action of this compound involves its interactions at the molecular level. It exerts its effects through binding interactions with biomolecules, enzyme inhibition or activation, and changes in gene expression . For example, in the process of enzymatic oxidation of methyl glycolate to this compound, the cascade catalysis of glycolate oxidase, catalase, and hemoglobin is involved .
Temporal Effects in Laboratory Settings
In laboratory settings, the effects of this compound change over time. Information on the product’s stability, degradation, and any long-term effects on cellular function observed in in vitro or in vivo studies is crucial . For instance, the fusion enzyme Vs HGB-GSG- So GOX mut -GGGGS- Hp CAT catalyzed the oxidation of 200 mM methyl glycolate for 6 h, giving a yield of 95.3% .
Metabolic Pathways
This compound is involved in several metabolic pathways. It is a key intermediate in the glyoxylate cycle, which is a variation of the tricarboxylic acid cycle . This cycle involves the conversion of acetyl-CoA to succinate for the synthesis of carbohydrates .
Transport and Distribution
This compound is transported and distributed within cells and tissues. It is usually generated and metabolized inside peroxisomes . The permeability of the peroxisomal membrane for small metabolites allows for the exchange of intermediates between proteins catalyzing consecutive reactions .
Subcellular Localization
The subcellular localization of this compound is primarily within the peroxisomes . This localization is crucial for its activity or function, as it is a key intermediate in the glyoxylate cycle, which occurs in the peroxisomes .
Preparation Methods
Synthetic Routes and Reaction Conditions
Methyl glyoxylate can be synthesized through the selective oxidation of methyl glycolate using molecular oxygen. This process involves the use of catalysts such as gold, palladium, and platinum supported on carriers like titanium dioxide, silicon dioxide, or zirconium dioxide. The reaction is typically carried out under mild conditions with oxygen or air as the oxidant .
Industrial Production Methods
In industrial settings, this compound is often produced as a by-product during the coal-to-ethylene glycol process. Methyl glycolate, an intermediate product of the hydrogenation of dimethyl oxalate to ethylene glycol, can be selectively oxidized to this compound. This method is advantageous due to its high conversion rate, selectivity, and environmentally friendly nature .
Chemical Reactions Analysis
Types of Reactions
Methyl glyoxylate undergoes various chemical reactions, including:
Oxidation: this compound can be further oxidized to glyoxylic acid.
Reduction: It can be reduced to methyl glycolate.
Substitution: The aldehyde group can undergo nucleophilic addition reactions.
Common Reagents and Conditions
Oxidation: Catalysts like gold, palladium, and platinum are used with molecular oxygen or air.
Reduction: Reducing agents such as sodium borohydride or lithium aluminum hydride are commonly used.
Substitution: Nucleophiles like amines or alcohols can react with the aldehyde group under mild conditions.
Major Products Formed
Oxidation: Glyoxylic acid.
Reduction: Methyl glycolate.
Substitution: Various substituted derivatives depending on the nucleophile used.
Scientific Research Applications
Methyl glyoxylate has numerous applications in scientific research, including:
Comparison with Similar Compounds
Similar Compounds
Glyoxylic acid: An aldehyde and carboxylic acid derivative similar to methyl glyoxylate.
Methyl glycolate: The precursor to this compound in oxidation reactions.
Glyoxal: A dialdehyde that shares structural similarities with this compound.
Uniqueness
This compound is unique due to its ester functional group, which imparts different reactivity compared to glyoxylic acid and glyoxal. Its ability to undergo selective oxidation and reduction reactions makes it a valuable intermediate in organic synthesis and industrial applications .
Properties
IUPAC Name |
methyl 2-oxoacetate | |
---|---|---|
Source | PubChem | |
URL | https://pubchem.ncbi.nlm.nih.gov | |
Description | Data deposited in or computed by PubChem | |
InChI |
InChI=1S/C3H4O3/c1-6-3(5)2-4/h2H,1H3 | |
Source | PubChem | |
URL | https://pubchem.ncbi.nlm.nih.gov | |
Description | Data deposited in or computed by PubChem | |
InChI Key |
KFKXSMSQHIOMSO-UHFFFAOYSA-N | |
Source | PubChem | |
URL | https://pubchem.ncbi.nlm.nih.gov | |
Description | Data deposited in or computed by PubChem | |
Canonical SMILES |
COC(=O)C=O | |
Source | PubChem | |
URL | https://pubchem.ncbi.nlm.nih.gov | |
Description | Data deposited in or computed by PubChem | |
Molecular Formula |
C3H4O3 | |
Source | PubChem | |
URL | https://pubchem.ncbi.nlm.nih.gov | |
Description | Data deposited in or computed by PubChem | |
Related CAS |
70205-93-5 | |
Record name | Acetic acid, 2-oxo-, methyl ester, homopolymer | |
Source | CAS Common Chemistry | |
URL | https://commonchemistry.cas.org/detail?cas_rn=70205-93-5 | |
Description | CAS Common Chemistry is an open community resource for accessing chemical information. Nearly 500,000 chemical substances from CAS REGISTRY cover areas of community interest, including common and frequently regulated chemicals, and those relevant to high school and undergraduate chemistry classes. This chemical information, curated by our expert scientists, is provided in alignment with our mission as a division of the American Chemical Society. | |
Explanation | The data from CAS Common Chemistry is provided under a CC-BY-NC 4.0 license, unless otherwise stated. | |
DSSTOX Substance ID |
DTXSID1052614 | |
Record name | Methyl oxoacetate | |
Source | EPA DSSTox | |
URL | https://comptox.epa.gov/dashboard/DTXSID1052614 | |
Description | DSSTox provides a high quality public chemistry resource for supporting improved predictive toxicology. | |
Molecular Weight |
88.06 g/mol | |
Source | PubChem | |
URL | https://pubchem.ncbi.nlm.nih.gov | |
Description | Data deposited in or computed by PubChem | |
CAS No. |
922-68-9 | |
Record name | Methyl glyoxylate | |
Source | CAS Common Chemistry | |
URL | https://commonchemistry.cas.org/detail?cas_rn=922-68-9 | |
Description | CAS Common Chemistry is an open community resource for accessing chemical information. Nearly 500,000 chemical substances from CAS REGISTRY cover areas of community interest, including common and frequently regulated chemicals, and those relevant to high school and undergraduate chemistry classes. This chemical information, curated by our expert scientists, is provided in alignment with our mission as a division of the American Chemical Society. | |
Explanation | The data from CAS Common Chemistry is provided under a CC-BY-NC 4.0 license, unless otherwise stated. | |
Record name | Acetic acid, 2-oxo-, methyl ester | |
Source | ChemIDplus | |
URL | https://pubchem.ncbi.nlm.nih.gov/substance/?source=chemidplus&sourceid=0000922689 | |
Description | ChemIDplus is a free, web search system that provides access to the structure and nomenclature authority files used for the identification of chemical substances cited in National Library of Medicine (NLM) databases, including the TOXNET system. | |
Record name | Acetic acid, 2-oxo-, methyl ester | |
Source | EPA Chemicals under the TSCA | |
URL | https://www.epa.gov/chemicals-under-tsca | |
Description | EPA Chemicals under the Toxic Substances Control Act (TSCA) collection contains information on chemicals and their regulations under TSCA, including non-confidential content from the TSCA Chemical Substance Inventory and Chemical Data Reporting. | |
Record name | Methyl oxoacetate | |
Source | EPA DSSTox | |
URL | https://comptox.epa.gov/dashboard/DTXSID1052614 | |
Description | DSSTox provides a high quality public chemistry resource for supporting improved predictive toxicology. | |
Record name | Methyl oxoacetate | |
Source | European Chemicals Agency (ECHA) | |
URL | https://echa.europa.eu/substance-information/-/substanceinfo/100.011.895 | |
Description | The European Chemicals Agency (ECHA) is an agency of the European Union which is the driving force among regulatory authorities in implementing the EU's groundbreaking chemicals legislation for the benefit of human health and the environment as well as for innovation and competitiveness. | |
Explanation | Use of the information, documents and data from the ECHA website is subject to the terms and conditions of this Legal Notice, and subject to other binding limitations provided for under applicable law, the information, documents and data made available on the ECHA website may be reproduced, distributed and/or used, totally or in part, for non-commercial purposes provided that ECHA is acknowledged as the source: "Source: European Chemicals Agency, http://echa.europa.eu/". Such acknowledgement must be included in each copy of the material. ECHA permits and encourages organisations and individuals to create links to the ECHA website under the following cumulative conditions: Links can only be made to webpages that provide a link to the Legal Notice page. | |
Record name | Methyl glyoxylate | |
Source | FDA Global Substance Registration System (GSRS) | |
URL | https://gsrs.ncats.nih.gov/ginas/app/beta/substances/A4JUE6B29X | |
Description | The FDA Global Substance Registration System (GSRS) enables the efficient and accurate exchange of information on what substances are in regulated products. Instead of relying on names, which vary across regulatory domains, countries, and regions, the GSRS knowledge base makes it possible for substances to be defined by standardized, scientific descriptions. | |
Explanation | Unless otherwise noted, the contents of the FDA website (www.fda.gov), both text and graphics, are not copyrighted. They are in the public domain and may be republished, reprinted and otherwise used freely by anyone without the need to obtain permission from FDA. Credit to the U.S. Food and Drug Administration as the source is appreciated but not required. | |
Retrosynthesis Analysis
AI-Powered Synthesis Planning: Our tool employs the Template_relevance Pistachio, Template_relevance Bkms_metabolic, Template_relevance Pistachio_ringbreaker, Template_relevance Reaxys, Template_relevance Reaxys_biocatalysis model, leveraging a vast database of chemical reactions to predict feasible synthetic routes.
One-Step Synthesis Focus: Specifically designed for one-step synthesis, it provides concise and direct routes for your target compounds, streamlining the synthesis process.
Accurate Predictions: Utilizing the extensive PISTACHIO, BKMS_METABOLIC, PISTACHIO_RINGBREAKER, REAXYS, REAXYS_BIOCATALYSIS database, our tool offers high-accuracy predictions, reflecting the latest in chemical research and data.
Strategy Settings
Precursor scoring | Relevance Heuristic |
---|---|
Min. plausibility | 0.01 |
Model | Template_relevance |
Template Set | Pistachio/Bkms_metabolic/Pistachio_ringbreaker/Reaxys/Reaxys_biocatalysis |
Top-N result to add to graph | 6 |
Feasible Synthetic Routes
Q1: What is the molecular formula and weight of methyl glyoxylate?
A: The molecular formula of this compound is C3H4O3, and its molecular weight is 88.06 g/mol. []
Q2: Is there any spectroscopic data available for this compound?
A: Yes, several studies use 1H and 13C NMR to characterize this compound and its derivatives. [, , , ] Additionally, FTIR spectroscopy has been used to study its degradation products in atmospheric reactions. []
Q3: this compound exists in equilibrium with its hydrate form. What factors influence this equilibrium?
A: The equilibrium between this compound and its hydrate form is affected by several factors, including the presence of water, methanol, and methyl glycolate. [] System pressure also plays a role in this equilibrium, influencing the volatility and reaction equilibrium of the system. []
Q4: What is known about the thermal stability of this compound polymers?
A: Poly(ethyl glyoxylate), a homologue of poly(this compound), exhibits enhanced thermal stability exceeding 200°C. [] The thermal stability is not significantly affected by molecular weight. []
Q5: How is this compound used in organic synthesis?
A: this compound serves as a versatile building block in organic synthesis. For example, it reacts with chloroacetyl chloride and triethylamine in the presence of a chiral imine derived from protected L-threonine to produce optically active α-amino acid derivatives with a cis-substituted β-lactam skeleton. []
Q6: Can this compound participate in cycloaddition reactions?
A: Yes, this compound and its derivatives are commonly used in cycloaddition reactions. For instance, this compound oxime undergoes both [3+2] and [4+2] cycloadditions with cyclopentadiene in the presence of Lewis or Bronsted acids. []
Q7: Have computational methods been employed to study reactions involving this compound?
A: Yes, DFT calculations have been used extensively to elucidate the mechanisms and stereoselectivity of reactions involving this compound and its derivatives. [, ] These studies provided insights into the role of Lewis acid catalysts and solvent effects on reaction pathways. [, ]
Q8: Has computational chemistry been used to study the degradation of methyl acrylate?
A: While the provided research doesn't directly utilize computational methods for methyl acrylate degradation, it highlights that its reaction with OH radicals primarily produces this compound and formaldehyde in the absence of NOx. [] This information could be used in future computational studies focusing on atmospheric chemistry models.
Q9: How is this compound prepared?
A: this compound can be synthesized through various methods. One approach involves an exchange reaction between dimethyl dimethoxyacetate and glyoxylic acid, followed by treatment with phosphorus pentoxide. [] Another method utilizes the oxidation of methyl glycolate to this compound, followed by hydrolysis. []
Q10: What are some significant reactions of this compound highlighted in the research?
A10: this compound engages in diverse reactions, including:
- Ene reaction: Reacts with α-lithiated this compound diethyl mercaptal to afford D-gluco-2-heptulosonate derivatives. []
- Condensation reaction: Reacts with o-aminobenzylpyrrole in a "Pictet-Spengler" type condensation to yield a tricyclic intermediate used in the synthesis of Aptazapine. []
- Cycloaddition reactions: Its oxime derivative undergoes [3+2] and [4+2] cycloadditions with dienes like cyclopentadiene. [, ]
- Formation of imines: Reacts with chiral amines to form imines, which can be used in asymmetric synthesis. [, , ]
Q11: What are some potential applications of this compound and its derivatives?
A11: this compound finds use in various applications, including:
- Synthesis of pharmaceuticals: Serves as a key building block in synthesizing bioactive molecules like β-lactam antibiotics [] and nucleoside analogues. []
- Chiral reagent: Its chiral derivatives, like L-methyl glyoxylate, are employed as chiral reagents in asymmetric synthesis. []
- Polymer chemistry: Used as a monomer in the production of biodegradable polymers, such as poly(this compound), which have potential applications in drug delivery and other fields. [, , ]
Q12: What happens to this compound in the atmosphere?
A: this compound is a product of the atmospheric degradation of methyl acrylate by OH radicals. [] Further studies are needed to understand its complete degradation pathway and environmental fate.
Q13: Is there research on the formation of this compound in astrophysical environments?
A: Yes, a study investigated the formation of organic residues in simulated interstellar conditions. It found that heating hydrogenated glyoxal ice between 10-300 K leads to solid residues. [] The analysis suggests these residues are likely a mixture of hydroxypyruvaldehyde and this compound. []
Disclaimer and Information on In-Vitro Research Products
Please be aware that all articles and product information presented on BenchChem are intended solely for informational purposes. The products available for purchase on BenchChem are specifically designed for in-vitro studies, which are conducted outside of living organisms. In-vitro studies, derived from the Latin term "in glass," involve experiments performed in controlled laboratory settings using cells or tissues. It is important to note that these products are not categorized as medicines or drugs, and they have not received approval from the FDA for the prevention, treatment, or cure of any medical condition, ailment, or disease. We must emphasize that any form of bodily introduction of these products into humans or animals is strictly prohibited by law. It is essential to adhere to these guidelines to ensure compliance with legal and ethical standards in research and experimentation.