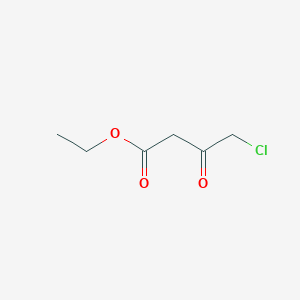
Ethyl 4-chloroacetoacetate
Overview
Description
Mechanism of Action
Target of Action
Ethyl 4-chloroacetoacetate is primarily used in the synthesis of phosphorus ylides . It is also used as a substrate in bioreduction processes, where it interacts with enzymes such as carbonyl reductase .
Mode of Action
The compound undergoes a highly stereoselective bioreduction process to transform into ®-ethyl 4-chloro-3-hydroxybutyrate . This process is facilitated by recombinant Escherichia coli containing carbonyl reductase and glucose dehydrogenase .
Biochemical Pathways
The bioreduction of this compound to ®-ethyl 4-chloro-3-hydroxybutyrate is a key step in the synthesis of certain pharmaceuticals . The process involves the reduction of the carbonyl group in the compound, resulting in the formation of a hydroxyl group .
Pharmacokinetics
Its transformation into ®-ethyl 4-chloro-3-hydroxybutyrate is known to occur at a ph of 70 and a temperature of 30°C . The kinetic constants Km and kcat of the compound were found to be 20.9 mM and 56.1 s−1, respectively .
Result of Action
The bioreduction of this compound results in the formation of ®-ethyl 4-chloro-3-hydroxybutyrate . This compound is a useful chiral building block for the synthesis of pharmaceuticals .
Action Environment
The bioreduction process is influenced by the presence of organic solvents and deep eutectic solvents . A high yield of ®-ethyl 4-chloro-3-hydroxybutyrate was achieved in an ethyl acetate-betaine/lactic acid-water system . Environmental factors such as pH and temperature also play a crucial role in the efficacy of the reaction .
Biochemical Analysis
Biochemical Properties
Ethyl 4-chloroacetoacetate plays a role in biochemical reactions, particularly in the synthesis of phosphorus ylides
Molecular Mechanism
It is known to be used in the synthesis of phosphorus ylides
Temporal Effects in Laboratory Settings
It is known that this compound has a boiling point of 115 °C .
Dosage Effects in Animal Models
It is known that this compound is toxic if swallowed and fatal in contact with skin .
Metabolic Pathways
It is known that this compound is used as an intermediate for organic syntheses .
Preparation Methods
Synthetic Routes and Reaction Conditions: The synthesis of Nolatrexed involves several steps. One method includes the Beckmann rearrangement of 3-bromo-4-methyl-alpha-oximinoacetanilide in strong sulfuric acid to obtain 4-bromo-5-methylisatin. This intermediate is then oxidized in a strong alkaline solution with hydrogen peroxide to yield 5-methyl-6-bromo-o-aminobenzoic acid. This compound is then cyclized with dicyandiamide in dilute sulfuric acid to form 2-guanidino-3,4-dihydro-5-bromo-6-methylquinazoline-4-ketone. The final step involves heating this intermediate with a strong base, 4-mercaptopyridine, and a nantokite catalyst to obtain Nolatrexed .
Industrial Production Methods: Industrial production of Nolatrexed typically follows similar synthetic routes but on a larger scale, with optimizations to improve yield and reduce costs. The process involves stringent control of reaction conditions to ensure the purity and efficacy of the final product .
Chemical Reactions Analysis
Types of Reactions: Nolatrexed undergoes various chemical reactions, including:
Oxidation: The intermediate 5-methyl-6-bromo-o-aminobenzoic acid is formed through oxidation with hydrogen peroxide.
Substitution: The final step in the synthesis involves a substitution reaction where 4-mercaptopyridine replaces a bromine atom.
Common Reagents and Conditions:
Oxidation: Hydrogen peroxide in a strong alkaline solution.
Substitution: 4-mercaptopyridine with a nantokite catalyst under reflux conditions.
Major Products: The major product of these reactions is Nolatrexed, with intermediates such as 4-bromo-5-methylisatin and 5-methyl-6-bromo-o-aminobenzoic acid formed along the way .
Scientific Research Applications
Nolatrexed has several scientific research applications:
Chemistry: Used as a model compound in studying thymidylate synthase inhibitors.
Biology: Investigated for its effects on cellular proliferation and DNA synthesis.
Medicine: Under clinical trials for treating various cancers, including liver cancer and head and neck cancer
Industry: Utilized in the development of new anticancer drugs and therapeutic agents.
Comparison with Similar Compounds
Properties
IUPAC Name |
ethyl 4-chloro-3-oxobutanoate | |
---|---|---|
Source | PubChem | |
URL | https://pubchem.ncbi.nlm.nih.gov | |
Description | Data deposited in or computed by PubChem | |
InChI |
InChI=1S/C6H9ClO3/c1-2-10-6(9)3-5(8)4-7/h2-4H2,1H3 | |
Source | PubChem | |
URL | https://pubchem.ncbi.nlm.nih.gov | |
Description | Data deposited in or computed by PubChem | |
InChI Key |
OHLRLMWUFVDREV-UHFFFAOYSA-N | |
Source | PubChem | |
URL | https://pubchem.ncbi.nlm.nih.gov | |
Description | Data deposited in or computed by PubChem | |
Canonical SMILES |
CCOC(=O)CC(=O)CCl | |
Source | PubChem | |
URL | https://pubchem.ncbi.nlm.nih.gov | |
Description | Data deposited in or computed by PubChem | |
Molecular Formula |
C6H9ClO3 | |
Source | PubChem | |
URL | https://pubchem.ncbi.nlm.nih.gov | |
Description | Data deposited in or computed by PubChem | |
DSSTOX Substance ID |
DTXSID4044454 | |
Record name | Ethyl 4-chloro-3-oxobutanoate | |
Source | EPA DSSTox | |
URL | https://comptox.epa.gov/dashboard/DTXSID4044454 | |
Description | DSSTox provides a high quality public chemistry resource for supporting improved predictive toxicology. | |
Molecular Weight |
164.59 g/mol | |
Source | PubChem | |
URL | https://pubchem.ncbi.nlm.nih.gov | |
Description | Data deposited in or computed by PubChem | |
CAS No. |
638-07-3 | |
Record name | Ethyl 4-chloroacetoacetate | |
Source | CAS Common Chemistry | |
URL | https://commonchemistry.cas.org/detail?cas_rn=638-07-3 | |
Description | CAS Common Chemistry is an open community resource for accessing chemical information. Nearly 500,000 chemical substances from CAS REGISTRY cover areas of community interest, including common and frequently regulated chemicals, and those relevant to high school and undergraduate chemistry classes. This chemical information, curated by our expert scientists, is provided in alignment with our mission as a division of the American Chemical Society. | |
Explanation | The data from CAS Common Chemistry is provided under a CC-BY-NC 4.0 license, unless otherwise stated. | |
Record name | Ethyl 4-chloro-3-oxobutanoate | |
Source | ChemIDplus | |
URL | https://pubchem.ncbi.nlm.nih.gov/substance/?source=chemidplus&sourceid=0000638073 | |
Description | ChemIDplus is a free, web search system that provides access to the structure and nomenclature authority files used for the identification of chemical substances cited in National Library of Medicine (NLM) databases, including the TOXNET system. | |
Record name | Butanoic acid, 4-chloro-3-oxo-, ethyl ester | |
Source | EPA Chemicals under the TSCA | |
URL | https://www.epa.gov/chemicals-under-tsca | |
Description | EPA Chemicals under the Toxic Substances Control Act (TSCA) collection contains information on chemicals and their regulations under TSCA, including non-confidential content from the TSCA Chemical Substance Inventory and Chemical Data Reporting. | |
Record name | Ethyl 4-chloro-3-oxobutanoate | |
Source | EPA DSSTox | |
URL | https://comptox.epa.gov/dashboard/DTXSID4044454 | |
Description | DSSTox provides a high quality public chemistry resource for supporting improved predictive toxicology. | |
Record name | Ethyl 4-chloroacetoacetate | |
Source | European Chemicals Agency (ECHA) | |
URL | https://echa.europa.eu/substance-information/-/substanceinfo/100.010.289 | |
Description | The European Chemicals Agency (ECHA) is an agency of the European Union which is the driving force among regulatory authorities in implementing the EU's groundbreaking chemicals legislation for the benefit of human health and the environment as well as for innovation and competitiveness. | |
Explanation | Use of the information, documents and data from the ECHA website is subject to the terms and conditions of this Legal Notice, and subject to other binding limitations provided for under applicable law, the information, documents and data made available on the ECHA website may be reproduced, distributed and/or used, totally or in part, for non-commercial purposes provided that ECHA is acknowledged as the source: "Source: European Chemicals Agency, http://echa.europa.eu/". Such acknowledgement must be included in each copy of the material. ECHA permits and encourages organisations and individuals to create links to the ECHA website under the following cumulative conditions: Links can only be made to webpages that provide a link to the Legal Notice page. | |
Record name | ETHYL 4-CHLOROACETOACETATE | |
Source | FDA Global Substance Registration System (GSRS) | |
URL | https://gsrs.ncats.nih.gov/ginas/app/beta/substances/5AE82250CM | |
Description | The FDA Global Substance Registration System (GSRS) enables the efficient and accurate exchange of information on what substances are in regulated products. Instead of relying on names, which vary across regulatory domains, countries, and regions, the GSRS knowledge base makes it possible for substances to be defined by standardized, scientific descriptions. | |
Explanation | Unless otherwise noted, the contents of the FDA website (www.fda.gov), both text and graphics, are not copyrighted. They are in the public domain and may be republished, reprinted and otherwise used freely by anyone without the need to obtain permission from FDA. Credit to the U.S. Food and Drug Administration as the source is appreciated but not required. | |
Synthesis routes and methods I
Procedure details
Synthesis routes and methods II
Procedure details
Synthesis routes and methods III
Procedure details
Retrosynthesis Analysis
AI-Powered Synthesis Planning: Our tool employs the Template_relevance Pistachio, Template_relevance Bkms_metabolic, Template_relevance Pistachio_ringbreaker, Template_relevance Reaxys, Template_relevance Reaxys_biocatalysis model, leveraging a vast database of chemical reactions to predict feasible synthetic routes.
One-Step Synthesis Focus: Specifically designed for one-step synthesis, it provides concise and direct routes for your target compounds, streamlining the synthesis process.
Accurate Predictions: Utilizing the extensive PISTACHIO, BKMS_METABOLIC, PISTACHIO_RINGBREAKER, REAXYS, REAXYS_BIOCATALYSIS database, our tool offers high-accuracy predictions, reflecting the latest in chemical research and data.
Strategy Settings
Precursor scoring | Relevance Heuristic |
---|---|
Min. plausibility | 0.01 |
Model | Template_relevance |
Template Set | Pistachio/Bkms_metabolic/Pistachio_ringbreaker/Reaxys/Reaxys_biocatalysis |
Top-N result to add to graph | 6 |
Feasible Synthetic Routes
Q1: What is the molecular formula and weight of ethyl 4-chloroacetoacetate?
A1: this compound has the molecular formula C6H9ClO3 and a molecular weight of 164.57 g/mol.
Q2: Is there any spectroscopic data available to confirm the structure of this compound?
A2: Yes, the structure of this compound and its derivatives has been confirmed using various spectroscopic techniques, including 1H NMR [, , , , ], 13C NMR [], 31P NMR [], IR [], and MS [].
Q3: What is the main application of this compound in organic synthesis?
A3: this compound is primarily used as a building block for synthesizing various heterocyclic compounds, including thiazoles [, , , ], thiadiazines [], pyrazoles [, ], imidazopyridines [, ], phthalides [], and pyrimidines [, , ].
Q4: Can you elaborate on the specific reactions this compound participates in?
A4: this compound readily undergoes reactions such as:
- Condensation reactions: It reacts with thiosemicarbazide to produce various thiazoles and thiadiazines under different reaction conditions []. It also condenses with aromatic aldehydes in Knoevenagel condensations, often carried out in ionic liquids [].
- Cyclization reactions: It participates in multicomponent reactions to yield complex heterocycles. For example, it reacts with phenyl isothiocyanate and active methylene reagents to form thiophene derivatives []. It can also be used in the synthesis of polysubstituted phthalides via a three-component cyclization reaction with aldehydes in the presence of piperidine, acid, and iodine [].
- Nucleophilic substitution: The chlorine atom can be replaced by various nucleophiles. For instance, it reacts with thiophenol to produce 4-phenylthio-ethyl acetoacetate via a sulfide etherification reaction [].
Q5: this compound is often employed in the synthesis of chiral compounds. What makes it suitable for this purpose?
A5: While this compound itself is not chiral, it serves as a precursor for chiral molecules. Its ketone functionality can be stereoselectively reduced to create a chiral center, leading to the formation of enantiomerically enriched alcohols [, , , , , , , , , , ].
Q6: Could you provide an example of how this compound is used in the synthesis of a specific pharmaceutical intermediate?
A6: this compound is a key starting material for the synthesis of ethyl (S)-4-chloro-3-hydroxybutanoate ((S)-ECHB) [, , , , , , , , ]. (S)-ECHB is a valuable chiral building block used in the production of pharmaceuticals, particularly statin drugs, which are widely prescribed for lowering cholesterol levels.
Q7: What is the role of biocatalysis in the context of this compound?
A7: Biocatalysis utilizes enzymes to facilitate chemical transformations. Several studies demonstrate the use of carbonyl reductases and alcohol dehydrogenases to catalyze the stereoselective reduction of this compound to produce enantiomerically pure (R)- or (S)-ethyl 4-chloro-3-hydroxybutanoate [, , , , , , , , , ].
Q8: Which microorganisms have been explored for the biocatalytic reduction of this compound?
A8: Several yeast species, including Aureobasidium pullulans [], Candida sorboxylosa [], Kluyveromyces aestuarii [], Kluyveromyces lactis [, ], Pichia capsulata [], Sporobolomyces salmonicolor [, ], and Zygosaccharomyces rouxii [, ] have been investigated for their ability to reduce this compound stereoselectively.
Q9: Are there any advantages to using biocatalysis over traditional chemical synthesis?
A9: Biocatalytic approaches often offer several advantages:* High enantioselectivity: Enzymes are highly specific catalysts, leading to the formation of products with high optical purity.* Mild reaction conditions: Biocatalytic reactions typically proceed under mild conditions (e.g., ambient temperature, neutral pH), which can be beneficial for labile substrates and reduce the formation of unwanted side products.* Environmentally friendly: Enzymes are biodegradable and often derived from renewable sources, making biocatalysis a more sustainable alternative to traditional chemical synthesis.
Q10: What can you tell me about the stability of this compound?
A10: The stability of this compound can be affected by various factors, including pH, temperature, and the presence of other chemicals. It's generally recommended to store it under cool and dry conditions. For example, one study highlighted that during the synthesis of 2-(2-aminothiazole-4yl)-2-(Z)-methoxyiminoacetic acid, a key intermediate derived from this compound, strict control over the reaction temperature was crucial to prevent unwanted side reactions and ensure high yields [].
Q11: Has computational chemistry been applied to the study of this compound?
A11: Yes, computational docking studies have been conducted to investigate the binding interactions of this compound and related substrates with various enzymes, including Aldo-keto reductases (AKRs) []. This type of analysis helps elucidate the substrate specificity of enzymes and guide the development of more efficient biocatalysts.
Disclaimer and Information on In-Vitro Research Products
Please be aware that all articles and product information presented on BenchChem are intended solely for informational purposes. The products available for purchase on BenchChem are specifically designed for in-vitro studies, which are conducted outside of living organisms. In-vitro studies, derived from the Latin term "in glass," involve experiments performed in controlled laboratory settings using cells or tissues. It is important to note that these products are not categorized as medicines or drugs, and they have not received approval from the FDA for the prevention, treatment, or cure of any medical condition, ailment, or disease. We must emphasize that any form of bodily introduction of these products into humans or animals is strictly prohibited by law. It is essential to adhere to these guidelines to ensure compliance with legal and ethical standards in research and experimentation.