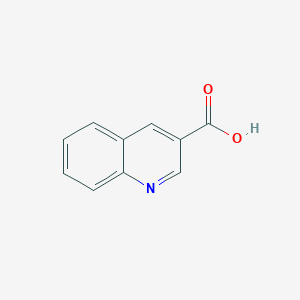
Quinoline-3-carboxylic acid
Overview
Description
Quinoline-3-carboxylic acid is a heterocyclic aromatic organic compound with a quinoline backbone. It is characterized by a carboxylic acid group attached to the third position of the quinoline ring. This compound is known for its diverse applications in medicinal chemistry, organic synthesis, and industrial processes.
Scientific Research Applications
Quinoline-3-carboxylic acid has a wide range of applications in scientific research:
Chemistry: It serves as a building block for the synthesis of complex organic molecules and heterocyclic compounds.
Biology: It is used in the study of enzyme inhibitors and receptor ligands.
Medicine: this compound derivatives exhibit antimicrobial, anticancer, and anti-inflammatory properties, making them valuable in drug development.
Industry: It is used in the production of dyes, pigments, and agrochemicals.
Mechanism of Action
Target of Action
Quinoline-3-carboxylic acid, a derivative of the quinoline compound, has been found to have significant biological activity. It is a vital scaffold for leads in drug discovery and plays a major role in the field of medicinal chemistry Quinoline derivatives have been reported to inhibit the proliferation of various cancer cells .
Mode of Action
Quinoline derivatives have been reported to exhibit their anticancer activities through the inhibition of tubulin polymerization, different kinases, topoisomerases, or by affecting dna cleavage activity .
Biochemical Pathways
Quinoline and its derivatives are known to affect various biological and pharmaceutical activities .
Pharmacokinetics
The pharmacokinetics of quinoline derivatives are an active area of research .
Result of Action
This compound has demonstrated significant antibacterial activity against various plant bacteria in vitro . Furthermore, it has shown a potent protective effect against Acidovorax citrulli, with an efficacy of 68.56% at 200 μg/mL . The treatment of Acidovorax citrulli cells with this compound resulted in a curved and sunken cell morphology, along with destroyed cell membrane integrity .
Action Environment
The action of this compound can be influenced by environmental factors. For instance, the unionized/nonpolar form in the acidic cancer tissue environment enhances the drug absorption ion in an acidic medium . This leads to better activity against cancer cells due to the minimal distribution of the drug in noncancerous cells .
Future Directions
Quinoline-3-carboxylic acid and its derivatives have shown potential for future drug development. They have been studied as inhibitors of protein kinase CK2 , and their interaction with DNA has been reported . The development of new effective inhibitors of CK2 is still actual . The recent in vivo and in vitro screening reported by scientists may pave the way for novel drug development . The derivatives of 3-quinoline carboxylic acid were found to inhibit CK2 with IC50 in the range from 0.65 to 18.2 μM . The most active inhibitors were found among tetrazolo-quinoline-4-carboxylic acid and 2-aminothis compound derivatives . These findings suggest that this compound and its derivatives could be promising candidates for the development of new drugs targeting neurodegenerative disorders .
Biochemical Analysis
Biochemical Properties
Quinoline-3-carboxylic acid has demonstrated numerous biological activities such as antimicrobial, insecticidal, anti-inflammatory, antiplatelet, and antitumor
Cellular Effects
This compound has shown significant activity against promastigotes and intracellular amastigotes, with less cytotoxicity compared to other compounds . It influences cell function, including impact on cell signaling pathways, gene expression, and cellular metabolism .
Molecular Mechanism
It is known to exert its effects at the molecular level, including potential binding interactions with biomolecules, possible enzyme inhibition or activation, and changes in gene expression .
Dosage Effects in Animal Models
The effects of this compound vary with different dosages in animal models
Metabolic Pathways
This compound is involved in the kynurenine pathway, which is responsible for the catabolism of tryptophan in mammals . It interacts with various enzymes or cofactors, and may have effects on metabolic flux or metabolite levels .
Transport and Distribution
It could interact with various transporters or binding proteins, and may have effects on its localization or accumulation .
Preparation Methods
Synthetic Routes and Reaction Conditions: Quinoline-3-carboxylic acid can be synthesized through various methods. One common approach involves the Skraup synthesis, which uses aniline, glycerol, and sulfuric acid as starting materials. Another method is the Doebner-Miller reaction, which involves the condensation of aniline with β-ketoesters under acidic conditions. Additionally, the Pfitzinger reaction, which involves the reaction of isatin with an aromatic amine in the presence of a base, can also be used to synthesize this compound .
Industrial Production Methods: Industrial production of this compound often employs catalytic processes to enhance yield and efficiency. Transition metal catalysts, such as palladium or copper, are commonly used in these processes. Green chemistry approaches, including the use of ionic liquids and microwave-assisted synthesis, have also been explored to minimize environmental impact .
Chemical Reactions Analysis
Types of Reactions: Quinoline-3-carboxylic acid undergoes various chemical reactions, including:
Oxidation: It can be oxidized to form this compound derivatives.
Reduction: Reduction reactions can convert it into this compound alcohols.
Substitution: Electrophilic and nucleophilic substitution reactions can introduce different functional groups into the quinoline ring.
Common Reagents and Conditions:
Oxidation: Common oxidizing agents include potassium permanganate and chromium trioxide.
Reduction: Reducing agents such as lithium aluminum hydride and sodium borohydride are used.
Substitution: Reagents like halogens, alkyl halides, and nitrating agents are employed under various conditions.
Major Products: The major products formed from these reactions include this compound derivatives with different functional groups, such as halogenated, alkylated, and nitrated quinolines .
Comparison with Similar Compounds
- Quinoline-2-carboxylic acid
- Quinoline-4-carboxylic acid
- Isoquinoline-3-carboxylic acid
Comparison: this compound is unique due to its specific position of the carboxylic acid group, which influences its chemical reactivity and biological activity. Compared to quinoline-2-carboxylic acid and quinoline-4-carboxylic acid, this compound exhibits distinct pharmacological properties and synthetic versatility .
Properties
IUPAC Name |
quinoline-3-carboxylic acid | |
---|---|---|
Source | PubChem | |
URL | https://pubchem.ncbi.nlm.nih.gov | |
Description | Data deposited in or computed by PubChem | |
InChI |
InChI=1S/C10H7NO2/c12-10(13)8-5-7-3-1-2-4-9(7)11-6-8/h1-6H,(H,12,13) | |
Source | PubChem | |
URL | https://pubchem.ncbi.nlm.nih.gov | |
Description | Data deposited in or computed by PubChem | |
InChI Key |
DJXNJVFEFSWHLY-UHFFFAOYSA-N | |
Source | PubChem | |
URL | https://pubchem.ncbi.nlm.nih.gov | |
Description | Data deposited in or computed by PubChem | |
Canonical SMILES |
C1=CC=C2C(=C1)C=C(C=N2)C(=O)O | |
Source | PubChem | |
URL | https://pubchem.ncbi.nlm.nih.gov | |
Description | Data deposited in or computed by PubChem | |
Molecular Formula |
C10H7NO2 | |
Source | PubChem | |
URL | https://pubchem.ncbi.nlm.nih.gov | |
Description | Data deposited in or computed by PubChem | |
DSSTOX Substance ID |
DTXSID90215101 | |
Record name | Quinoline-3-carboxylic acid | |
Source | EPA DSSTox | |
URL | https://comptox.epa.gov/dashboard/DTXSID90215101 | |
Description | DSSTox provides a high quality public chemistry resource for supporting improved predictive toxicology. | |
Molecular Weight |
173.17 g/mol | |
Source | PubChem | |
URL | https://pubchem.ncbi.nlm.nih.gov | |
Description | Data deposited in or computed by PubChem | |
CAS No. |
6480-68-8 | |
Record name | 3-Quinolinecarboxylic acid | |
Source | CAS Common Chemistry | |
URL | https://commonchemistry.cas.org/detail?cas_rn=6480-68-8 | |
Description | CAS Common Chemistry is an open community resource for accessing chemical information. Nearly 500,000 chemical substances from CAS REGISTRY cover areas of community interest, including common and frequently regulated chemicals, and those relevant to high school and undergraduate chemistry classes. This chemical information, curated by our expert scientists, is provided in alignment with our mission as a division of the American Chemical Society. | |
Explanation | The data from CAS Common Chemistry is provided under a CC-BY-NC 4.0 license, unless otherwise stated. | |
Record name | Quinoline-3-carboxylic acid | |
Source | ChemIDplus | |
URL | https://pubchem.ncbi.nlm.nih.gov/substance/?source=chemidplus&sourceid=0006480688 | |
Description | ChemIDplus is a free, web search system that provides access to the structure and nomenclature authority files used for the identification of chemical substances cited in National Library of Medicine (NLM) databases, including the TOXNET system. | |
Record name | 3-Quinolinecarboxylic acid | |
Source | DTP/NCI | |
URL | https://dtp.cancer.gov/dtpstandard/servlet/dwindex?searchtype=NSC&outputformat=html&searchlist=403263 | |
Description | The NCI Development Therapeutics Program (DTP) provides services and resources to the academic and private-sector research communities worldwide to facilitate the discovery and development of new cancer therapeutic agents. | |
Explanation | Unless otherwise indicated, all text within NCI products is free of copyright and may be reused without our permission. Credit the National Cancer Institute as the source. | |
Record name | Quinoline-3-carboxylic acid | |
Source | EPA DSSTox | |
URL | https://comptox.epa.gov/dashboard/DTXSID90215101 | |
Description | DSSTox provides a high quality public chemistry resource for supporting improved predictive toxicology. | |
Record name | Quinoline-3-carboxylic acid | |
Source | European Chemicals Agency (ECHA) | |
URL | https://echa.europa.eu/substance-information/-/substanceinfo/100.026.671 | |
Description | The European Chemicals Agency (ECHA) is an agency of the European Union which is the driving force among regulatory authorities in implementing the EU's groundbreaking chemicals legislation for the benefit of human health and the environment as well as for innovation and competitiveness. | |
Explanation | Use of the information, documents and data from the ECHA website is subject to the terms and conditions of this Legal Notice, and subject to other binding limitations provided for under applicable law, the information, documents and data made available on the ECHA website may be reproduced, distributed and/or used, totally or in part, for non-commercial purposes provided that ECHA is acknowledged as the source: "Source: European Chemicals Agency, http://echa.europa.eu/". Such acknowledgement must be included in each copy of the material. ECHA permits and encourages organisations and individuals to create links to the ECHA website under the following cumulative conditions: Links can only be made to webpages that provide a link to the Legal Notice page. | |
Record name | QUINOLINE-3-CARBOXYLIC ACID | |
Source | FDA Global Substance Registration System (GSRS) | |
URL | https://gsrs.ncats.nih.gov/ginas/app/beta/substances/D98JB7TM7H | |
Description | The FDA Global Substance Registration System (GSRS) enables the efficient and accurate exchange of information on what substances are in regulated products. Instead of relying on names, which vary across regulatory domains, countries, and regions, the GSRS knowledge base makes it possible for substances to be defined by standardized, scientific descriptions. | |
Explanation | Unless otherwise noted, the contents of the FDA website (www.fda.gov), both text and graphics, are not copyrighted. They are in the public domain and may be republished, reprinted and otherwise used freely by anyone without the need to obtain permission from FDA. Credit to the U.S. Food and Drug Administration as the source is appreciated but not required. | |
Synthesis routes and methods
Procedure details
Retrosynthesis Analysis
AI-Powered Synthesis Planning: Our tool employs the Template_relevance Pistachio, Template_relevance Bkms_metabolic, Template_relevance Pistachio_ringbreaker, Template_relevance Reaxys, Template_relevance Reaxys_biocatalysis model, leveraging a vast database of chemical reactions to predict feasible synthetic routes.
One-Step Synthesis Focus: Specifically designed for one-step synthesis, it provides concise and direct routes for your target compounds, streamlining the synthesis process.
Accurate Predictions: Utilizing the extensive PISTACHIO, BKMS_METABOLIC, PISTACHIO_RINGBREAKER, REAXYS, REAXYS_BIOCATALYSIS database, our tool offers high-accuracy predictions, reflecting the latest in chemical research and data.
Strategy Settings
Precursor scoring | Relevance Heuristic |
---|---|
Min. plausibility | 0.01 |
Model | Template_relevance |
Template Set | Pistachio/Bkms_metabolic/Pistachio_ringbreaker/Reaxys/Reaxys_biocatalysis |
Top-N result to add to graph | 6 |
Feasible Synthetic Routes
Q1: What is the primary mechanism of action for quinoline-3-carboxylic acid derivatives as antibacterial agents?
A: this compound derivatives, often referred to as quinolones, exert their antibacterial effects primarily by inhibiting bacterial enzymes essential for DNA replication. These enzymes, DNA gyrase and topoisomerase IV, are crucial for maintaining the topological state of DNA, which is necessary for bacterial survival and proliferation. []
Q2: How do quinolones interact with DNA gyrase and topoisomerase IV?
A: Quinolones bind to the enzyme-DNA complex, forming a stable ternary complex that disrupts DNA replication. This interaction leads to the accumulation of double-stranded DNA breaks, ultimately causing bacterial cell death. []
Q3: Are there structural differences in the way quinolones interact with DNA gyrase and topoisomerase IV?
A: Yes, while both enzymes are targeted, certain structural features of quinolones can influence their binding affinity for one enzyme over the other. For instance, besifloxacin exhibits a more balanced activity against both enzymes compared to other fluoroquinolones. []
Q4: What is the significance of the C-7 substituent in quinolone antibacterial activity?
A: The C-7 substituent plays a crucial role in enhancing antibacterial activity. Studies indicate that a 2-aminoethylthio group at C-7 significantly improves in vitro antibacterial activity. []
Q5: How do C-5 substituents affect the antibacterial potency of quinolones?
A: Modifications at the C-5 position also impact activity. Research suggests the following order of increasing activity based on C-5 substituents: OH < F < H < NH2. []
Q6: Are there quinolone derivatives with activity against drug-resistant bacteria?
A: Yes, certain quinolone derivatives show promise against drug-resistant strains. For instance, compounds like 7-substituted-6-fluoro-1-fluoromethyl-4-oxo-4H-[1,3]thiazeto[3,2-a]this compound derivatives (2a-1) have demonstrated excellent in vitro and in vivo activity against both gram-negative and gram-positive bacteria, including quinolone-resistant and Methicillin-resistant Staphylococcus aureus. []
Q7: What is the molecular formula of a simple this compound?
A7: The molecular formula for the unsubstituted this compound is C10H7NO2.
Q8: How are substituted this compound derivatives typically synthesized?
A: Various synthetic strategies have been explored, often involving multi-step reactions. For example, the synthesis of 6,7-dihalogen-N-ethyl-4-oxo-l, 4-dihydroquinoline-3-carboxylic acids (3a, b) begins with 3,4-dihalogen-anilines and proceeds through N-ethylation, reaction with ortho formic ester and Meldrum's acid, and ultimately, intramolecular cyclization. []
Q9: Can you provide an example of a one-pot synthesis for specific this compound derivatives?
A: Yes, a one-pot three-step synthesis has been reported for 2-(1-benzofuran-2-yl)this compound derivatives. This efficient method involves a Williamson ether synthesis, hydrolysis of an ester group at the quinoline ring C-3 position, and finally, an intramolecular electrophilic cyclization reaction. []
Q10: How do structural modifications influence the biological activity of quinolone-3-carboxylic acid derivatives?
A10: SAR studies reveal that even subtle structural changes significantly impact a quinolone's potency, spectrum of activity, and potential for resistance development.
Q11: What are some critical structural modifications that impact the antibacterial activity of quinolones?
A: Substitutions at positions C-6, C-7, and C-8, particularly with fluorine or other halogens, often enhance activity against Gram-negative bacteria. The nature of the substituent at N-1 also affects activity, with cyclopropyl groups being frequently incorporated. [, ]
Q12: What are some examples of substituents at the C-7 position and their effects?
A: Various groups, including piperazine, pyrrolidine, and substituted amine moieties, have been explored at C-7. These modifications modulate not only antibacterial potency but also pharmacokinetic properties. For instance, a piperazinyl group at C-7 is found in many clinically relevant quinolones. [, , ]
Q13: Are quinolone-3-carboxylic acid derivatives susceptible to photodegradation?
A: Yes, like many pharmaceuticals, these compounds can degrade upon exposure to light. For example, besifloxacin undergoes photodegradation under both aerobic and anaerobic conditions when irradiated with UV-A light. [, ]
Q14: What are the potential consequences of photodegradation for quinolone-containing medications?
A: Photodegradation can lead to the formation of less active or potentially toxic byproducts. It also compromises the stability and effectiveness of the drug formulation. []
Q15: What is known about the pharmacokinetics of quinolone-3-carboxylic acid derivatives?
A: Pharmacokinetic properties vary widely depending on the specific substituents present. Factors such as lipophilicity, ionization state, and molecular weight contribute to their absorption, distribution, metabolism, and excretion profiles. []
Q16: Do quinolones exhibit interactions with drug transporters?
A: Yes, some quinolones interact with drug transporters, particularly those belonging to the ATP-binding cassette (ABC) transporter family. These interactions can affect the absorption, distribution, and elimination of quinolones, potentially leading to drug-drug interactions or altered pharmacokinetic profiles. []
Q17: How does bacterial resistance to quinolone-3-carboxylic acid derivatives develop?
A: Resistance to quinolones can arise through various mechanisms, including mutations in the genes encoding DNA gyrase and topoisomerase IV, reducing drug binding affinity. Other mechanisms include increased efflux of the drug from the bacterial cell, reduced drug uptake, and protection of the target enzymes by other proteins. []
Q18: Is there cross-resistance between quinolones and other classes of antibacterial agents?
A: While cross-resistance between quinolones and other classes like β-lactams is not generally observed, it can occur with agents that share similar mechanisms of action or resistance determinants. For instance, the use of one quinolone might select for bacteria with mutations that also confer resistance to other quinolones. []
Q19: Are there specific patient populations where quinolone use requires caution?
A: Yes, caution is advised in patients with a history of seizures, as quinolones can lower the seizure threshold. They should also be used with caution in patients with known or suspected glucose-6-phosphate dehydrogenase (G6PD) deficiency, as they may precipitate hemolysis. []
Q20: Beyond their antibacterial properties, are there other potential applications for this compound derivatives?
A: Yes, research suggests that quinoline derivatives hold promise in various therapeutic areas, including antiviral, anticancer, and anti-inflammatory applications. [, ]
Q21: Can you elaborate on the potential of quinolones as antiviral agents?
A: Certain quinoline derivatives have demonstrated inhibitory activity against viral enzymes like HIV-1 reverse transcriptase. For example, 6-chloro-1,4-dihydro-4-oxo-1-(beta-D-ribofuranosyl)-quinoline-3-carboxylic acid (compound A) shows promise as an HIV-1 inhibitor by targeting the enzyme reverse transcriptase. []
Q22: What are some key challenges in developing new quinolone-based drugs?
A: Challenges include overcoming existing bacterial resistance mechanisms, mitigating potential toxicity concerns, and optimizing pharmacokinetic properties for improved efficacy and safety profiles. []
Disclaimer and Information on In-Vitro Research Products
Please be aware that all articles and product information presented on BenchChem are intended solely for informational purposes. The products available for purchase on BenchChem are specifically designed for in-vitro studies, which are conducted outside of living organisms. In-vitro studies, derived from the Latin term "in glass," involve experiments performed in controlled laboratory settings using cells or tissues. It is important to note that these products are not categorized as medicines or drugs, and they have not received approval from the FDA for the prevention, treatment, or cure of any medical condition, ailment, or disease. We must emphasize that any form of bodily introduction of these products into humans or animals is strictly prohibited by law. It is essential to adhere to these guidelines to ensure compliance with legal and ethical standards in research and experimentation.