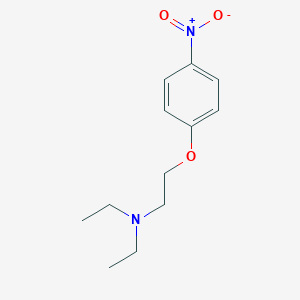
N,N-diethyl-2-(4-nitrophenoxy)ethanamine
Overview
Description
N,N-diethyl-2-(4-nitrophenoxy)ethanamine: is an organic compound with the molecular formula C12H18N2O3 It is characterized by the presence of a nitrophenoxy group attached to an ethanamine backbone, with diethyl groups on the nitrogen atom
Preparation Methods
Synthetic Routes and Reaction Conditions:
Starting Materials: The synthesis typically begins with 4-nitrophenol and N,N-diethylethanolamine.
Reaction Steps:
Reaction Conditions: The reactions are typically carried out in an inert atmosphere, such as nitrogen, and may require heating to facilitate the reactions.
Industrial Production Methods: The industrial production of N,N-diethyl-2-(4-nitrophenoxy)ethanamine follows similar synthetic routes but on a larger scale. The process involves:
Bulk Handling: Large quantities of starting materials are handled using automated systems.
Reactor Design: Specialized reactors that can maintain the required temperature and pressure conditions are used.
Purification: The product is purified using techniques such as distillation or crystallization to achieve the desired purity.
Chemical Reactions Analysis
Types of Reactions:
Oxidation: The nitro group in N,N-diethyl-2-(4-nitrophenoxy)ethanamine can undergo reduction to form the corresponding amine.
Reduction: The compound can be reduced using reducing agents like hydrogen gas in the presence of a catalyst.
Substitution: The ethanamine group can participate in nucleophilic substitution reactions.
Common Reagents and Conditions:
Oxidation: Reagents like potassium permanganate or chromium trioxide can be used.
Reduction: Common reducing agents include hydrogen gas with a palladium catalyst or lithium aluminum hydride.
Substitution: Nucleophiles such as alkyl halides or acyl chlorides can be used under basic conditions.
Major Products Formed:
Reduction Product: 2-(4-aminophenoxy)ethanamine.
Substitution Products: Various substituted ethanamines depending on the nucleophile used.
Scientific Research Applications
Chemistry:
Synthesis of Derivatives: N,N-diethyl-2-(4-nitrophenoxy)ethanamine is used as an intermediate in the synthesis of various organic compounds.
Catalysis: It can act as a ligand in catalytic reactions.
Biology:
Biochemical Studies: The compound is used in studies involving enzyme interactions and inhibition.
Medicine:
Pharmaceuticals: It serves as a precursor in the synthesis of drugs with potential therapeutic effects.
Industry:
Material Science: The compound is used in the development of new materials with specific properties.
Mechanism of Action
The mechanism of action of N,N-diethyl-2-(4-nitrophenoxy)ethanamine involves its interaction with molecular targets such as enzymes or receptors. The nitrophenoxy group can participate in hydrogen bonding and electrostatic interactions, while the ethanamine backbone provides structural flexibility. These interactions can modulate the activity of the target molecules, leading to various biological effects.
Comparison with Similar Compounds
- N,N-dimethyl-2-(4-nitrophenoxy)ethanamine
- N,N-diethyl-2-(4-aminophenoxy)ethanamine
- N,N-diethyl-2-(4-chlorophenoxy)ethanamine
Comparison:
- N,N-dimethyl-2-(4-nitrophenoxy)ethanamine: Similar structure but with methyl groups instead of ethyl groups, leading to different steric and electronic properties.
- N,N-diethyl-2-(4-aminophenoxy)ethanamine: The nitro group is reduced to an amine, altering its reactivity and potential applications.
- N,N-diethyl-2-(4-chlorophenoxy)ethanamine: The nitro group is replaced with a chloro group, affecting its chemical behavior and interactions.
N,N-diethyl-2-(4-nitrophenoxy)ethanamine stands out due to its unique combination of a nitrophenoxy group and an ethanamine backbone, providing a balance of reactivity and stability that is valuable in various applications.
Biological Activity
N,N-Diethyl-2-(4-nitrophenoxy)ethanamine is a compound that has garnered attention in scientific research due to its potential biological activities and applications in medicinal chemistry. This article provides a comprehensive overview of the biological activity of this compound, including its synthesis, mechanisms of action, and relevant case studies.
Chemical Structure and Properties
This compound has the following chemical structure:
- Molecular Formula : CHNO
- Molecular Weight : Approximately 226.29 g/mol
The compound features a diethylamino group and a 4-nitrophenoxy moiety, which contribute to its unique chemical reactivity and biological interactions.
Synthesis Methods
The synthesis of this compound can be achieved through various methods, typically involving nucleophilic substitution reactions. A common synthetic route includes:
- Starting Materials : 4-nitrophenol and diethylamine.
- Reagents : Use of a suitable base (e.g., sodium hydroxide) to facilitate the reaction.
- Procedure : The reaction mixture is stirred under controlled temperature conditions to yield the desired product.
The biological activity of this compound is primarily attributed to its ability to interact with specific molecular targets within biological systems. The nitrophenoxy group can participate in electron transfer reactions, while the ethanamine backbone may interact with various receptors and enzymes, modulating biochemical pathways.
Key Mechanisms:
- Inhibition of Kinase Pathways : Research suggests that this compound may inhibit specific kinase pathways, such as the phosphoinositide 3-kinase (PI3K) pathway, which is crucial for cell growth and survival.
- Effects on Cell Proliferation : Studies indicate that this compound may affect cell proliferation rates in cancer cell lines, demonstrating potential as an anticancer agent.
Biological Activity and Research Findings
This compound has been investigated for various biological activities, particularly in the context of cancer research.
Anticancer Activity
Recent studies have highlighted its potential effectiveness against several cancer types:
- Breast Cancer : Exhibited significant cytotoxic effects on breast cancer cell lines (IC values indicating effective concentrations).
- Prostate Cancer : Similar inhibitory effects have been observed in prostate cancer models.
Cancer Type | Cell Line | IC (µM) |
---|---|---|
Breast | T47D | 12.5 |
Prostate | LNCaP | 15.0 |
Case Studies
- Cell-Based Assays : In vitro studies using cell lines have demonstrated that treatment with this compound leads to increased apoptosis rates compared to untreated controls.
- Mechanistic Studies : Investigations into the compound's mechanism revealed that it may induce autophagy in cancer cells, contributing to its anticancer properties.
Comparative Analysis with Similar Compounds
This compound shares structural similarities with other compounds in its class, which also exhibit notable biological activities:
Compound Name | Molecular Formula | Key Features |
---|---|---|
N,N-Dimethyl-2-(4-nitrophenoxy)ethanamine | CHNO | Contains dimethyl groups instead of diethyl |
N,N-Diethyl-2-(3-nitrophenoxy)ethanamine | CHNO | Nitro group positioned at the meta position |
These comparisons highlight how variations in substituents can influence biological activity and therapeutic potential.
Properties
IUPAC Name |
N,N-diethyl-2-(4-nitrophenoxy)ethanamine | |
---|---|---|
Source | PubChem | |
URL | https://pubchem.ncbi.nlm.nih.gov | |
Description | Data deposited in or computed by PubChem | |
InChI |
InChI=1S/C12H18N2O3/c1-3-13(4-2)9-10-17-12-7-5-11(6-8-12)14(15)16/h5-8H,3-4,9-10H2,1-2H3 | |
Source | PubChem | |
URL | https://pubchem.ncbi.nlm.nih.gov | |
Description | Data deposited in or computed by PubChem | |
InChI Key |
ZLZZAXUKBHFTHA-UHFFFAOYSA-N | |
Source | PubChem | |
URL | https://pubchem.ncbi.nlm.nih.gov | |
Description | Data deposited in or computed by PubChem | |
Canonical SMILES |
CCN(CC)CCOC1=CC=C(C=C1)[N+](=O)[O-] | |
Source | PubChem | |
URL | https://pubchem.ncbi.nlm.nih.gov | |
Description | Data deposited in or computed by PubChem | |
Molecular Formula |
C12H18N2O3 | |
Source | PubChem | |
URL | https://pubchem.ncbi.nlm.nih.gov | |
Description | Data deposited in or computed by PubChem | |
DSSTOX Substance ID |
DTXSID10173638 | |
Record name | Diethyl(2-(4-nitrophenoxy)ethyl)amine | |
Source | EPA DSSTox | |
URL | https://comptox.epa.gov/dashboard/DTXSID10173638 | |
Description | DSSTox provides a high quality public chemistry resource for supporting improved predictive toxicology. | |
Molecular Weight |
238.28 g/mol | |
Source | PubChem | |
URL | https://pubchem.ncbi.nlm.nih.gov | |
Description | Data deposited in or computed by PubChem | |
CAS No. |
19881-36-8 | |
Record name | N,N-Diethyl-2-(4-nitrophenoxy)ethanamine | |
Source | CAS Common Chemistry | |
URL | https://commonchemistry.cas.org/detail?cas_rn=19881-36-8 | |
Description | CAS Common Chemistry is an open community resource for accessing chemical information. Nearly 500,000 chemical substances from CAS REGISTRY cover areas of community interest, including common and frequently regulated chemicals, and those relevant to high school and undergraduate chemistry classes. This chemical information, curated by our expert scientists, is provided in alignment with our mission as a division of the American Chemical Society. | |
Explanation | The data from CAS Common Chemistry is provided under a CC-BY-NC 4.0 license, unless otherwise stated. | |
Record name | Diethyl(2-(4-nitrophenoxy)ethyl)amine | |
Source | ChemIDplus | |
URL | https://pubchem.ncbi.nlm.nih.gov/substance/?source=chemidplus&sourceid=0019881368 | |
Description | ChemIDplus is a free, web search system that provides access to the structure and nomenclature authority files used for the identification of chemical substances cited in National Library of Medicine (NLM) databases, including the TOXNET system. | |
Record name | Diethyl(2-(4-nitrophenoxy)ethyl)amine | |
Source | EPA DSSTox | |
URL | https://comptox.epa.gov/dashboard/DTXSID10173638 | |
Description | DSSTox provides a high quality public chemistry resource for supporting improved predictive toxicology. | |
Record name | Diethyl[2-(4-nitrophenoxy)ethyl]amine | |
Source | European Chemicals Agency (ECHA) | |
URL | https://echa.europa.eu/substance-information/-/substanceinfo/100.039.434 | |
Description | The European Chemicals Agency (ECHA) is an agency of the European Union which is the driving force among regulatory authorities in implementing the EU's groundbreaking chemicals legislation for the benefit of human health and the environment as well as for innovation and competitiveness. | |
Explanation | Use of the information, documents and data from the ECHA website is subject to the terms and conditions of this Legal Notice, and subject to other binding limitations provided for under applicable law, the information, documents and data made available on the ECHA website may be reproduced, distributed and/or used, totally or in part, for non-commercial purposes provided that ECHA is acknowledged as the source: "Source: European Chemicals Agency, http://echa.europa.eu/". Such acknowledgement must be included in each copy of the material. ECHA permits and encourages organisations and individuals to create links to the ECHA website under the following cumulative conditions: Links can only be made to webpages that provide a link to the Legal Notice page. | |
Synthesis routes and methods I
Procedure details
Synthesis routes and methods II
Procedure details
Synthesis routes and methods III
Procedure details
Synthesis routes and methods IV
Procedure details
Synthesis routes and methods V
Procedure details
Retrosynthesis Analysis
AI-Powered Synthesis Planning: Our tool employs the Template_relevance Pistachio, Template_relevance Bkms_metabolic, Template_relevance Pistachio_ringbreaker, Template_relevance Reaxys, Template_relevance Reaxys_biocatalysis model, leveraging a vast database of chemical reactions to predict feasible synthetic routes.
One-Step Synthesis Focus: Specifically designed for one-step synthesis, it provides concise and direct routes for your target compounds, streamlining the synthesis process.
Accurate Predictions: Utilizing the extensive PISTACHIO, BKMS_METABOLIC, PISTACHIO_RINGBREAKER, REAXYS, REAXYS_BIOCATALYSIS database, our tool offers high-accuracy predictions, reflecting the latest in chemical research and data.
Strategy Settings
Precursor scoring | Relevance Heuristic |
---|---|
Min. plausibility | 0.01 |
Model | Template_relevance |
Template Set | Pistachio/Bkms_metabolic/Pistachio_ringbreaker/Reaxys/Reaxys_biocatalysis |
Top-N result to add to graph | 6 |
Feasible Synthetic Routes
Disclaimer and Information on In-Vitro Research Products
Please be aware that all articles and product information presented on BenchChem are intended solely for informational purposes. The products available for purchase on BenchChem are specifically designed for in-vitro studies, which are conducted outside of living organisms. In-vitro studies, derived from the Latin term "in glass," involve experiments performed in controlled laboratory settings using cells or tissues. It is important to note that these products are not categorized as medicines or drugs, and they have not received approval from the FDA for the prevention, treatment, or cure of any medical condition, ailment, or disease. We must emphasize that any form of bodily introduction of these products into humans or animals is strictly prohibited by law. It is essential to adhere to these guidelines to ensure compliance with legal and ethical standards in research and experimentation.