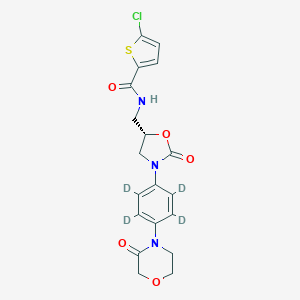
Rivaroxaban-d4
Overview
Description
Rivaroxaban-d4 is a deuterated form of rivaroxaban, an oral anticoagulant that directly inhibits Factor Xa. It is primarily used as an internal standard for the quantification of rivaroxaban in various analytical methods, such as gas chromatography-mass spectrometry (GC-MS) and liquid chromatography-mass spectrometry (LC-MS) . The deuterium atoms in this compound replace hydrogen atoms, which helps in distinguishing it from the non-deuterated form during analysis.
Scientific Research Applications
Rivaroxaban-d4 is widely used in scientific research for various applications, including:
Chemistry: Used as an internal standard in analytical chemistry for the quantification of rivaroxaban.
Biology: Employed in studies involving the metabolism and pharmacokinetics of rivaroxaban.
Medicine: Utilized in clinical research to monitor the levels of rivaroxaban in patients.
Industry: Applied in the development and quality control of pharmaceutical formulations containing rivaroxaban.
Mechanism of Action
Target of Action
Rivaroxaban-d4, like its parent compound Rivaroxaban, is a potent and selective inhibitor of Factor Xa (FXa), a crucial component of the intrinsic and extrinsic pathways of the blood coagulation cascade . Factor Xa plays a central role in blood coagulation, responsible for converting prothrombin (Factor II) to thrombin (Factor IIa) .
Mode of Action
This compound competitively inhibits free and clot-bound Factor Xa . By blocking the activity of Factor Xa, this compound prevents the conversion of prothrombin to thrombin, thereby inhibiting the formation of fibrin clots . This action disrupts the coagulation cascade, preventing the formation of blood clots.
Biochemical Pathways
The primary biochemical pathway affected by this compound is the coagulation cascade. By inhibiting Factor Xa, this compound disrupts the conversion of prothrombin to thrombin, a key step in the coagulation cascade . This inhibition prevents the formation of fibrin clots, thereby exerting its anticoagulant effect .
Pharmacokinetics
This compound, like Rivaroxaban, is rapidly absorbed with high oral bioavailability . The pharmacokinetics of Rivaroxaban is well described by an oral one-compartment model . The estimated apparent clearance (CL/F) and volume of distribution (V/F) are influenced by demographic factors such as age, renal function, body weight, and gender . The elimination half-life of Rivaroxaban is 5-9 hours in healthy young subjects and 11-13 hours in elderly subjects .
Result of Action
The primary result of this compound’s action is the prevention of blood clot formation. By inhibiting Factor Xa, this compound prevents the formation of fibrin clots, thereby reducing the risk of thromboembolic events such as deep vein thrombosis (DVT) and pulmonary embolism (PE) . It is also used for stroke prevention in patients with non-valvular atrial fibrillation .
Action Environment
The action of this compound can be influenced by various environmental factors. For instance, the bioavailability of Rivaroxaban is affected by food intake . Additionally, Rivaroxaban’s clearance and volume of distribution can be influenced by factors such as age, renal function, body weight, and gender . Genetic polymorphisms may also influence the pharmacokinetics and pharmacodynamics of Rivaroxaban .
Safety and Hazards
Future Directions
The FDA has approved two pediatric indications for Rivaroxaban: the treatment of venous thromboembolism (VTE, or blood clots) and reduction in the risk of recurrent VTE in patients from birth to less than 18 years after at least five days of initial parenteral (injected or intravenous) anticoagulant treatment; and thromboprophylaxis (prevention of blood clots and blood-clot related events) in children aged two years and older with congenital heart disease who have undergone the Fontan procedure . This suggests that Rivaroxaban and its derivatives like Rivaroxaban-d4 may have more applications in the future.
Biochemical Analysis
Biochemical Properties
Rivaroxaban-d4 demonstrates a significant selectivity for Factor Xa, which is over 10,000-fold greater compared to other related serine proteases . Factor Xa is a key enzyme in the coagulation cascade, playing a central role in thrombin formation . This compound, by inhibiting Factor Xa, interferes with the normal clotting process, making it a potent anticoagulant .
Cellular Effects
This compound, like its parent compound rivaroxaban, exerts its effects at the cellular level primarily by inhibiting Factor Xa . This inhibition disrupts the coagulation cascade, reducing thrombin formation and thereby decreasing the likelihood of blood clot formation .
Molecular Mechanism
This compound acts as a direct inhibitor of Factor Xa . It binds directly and reversibly to Factor Xa, inhibiting both free and clot-bound Factor Xa, as well as prothrombinase activity . This inhibition disrupts the conversion of prothrombin to thrombin, a crucial step in the coagulation cascade .
Temporal Effects in Laboratory Settings
The pharmacokinetic profile of rivaroxaban, which this compound is used to quantify, is consistent across a broad range of patient populations . It is absorbed rapidly, with maximum plasma concentrations being reached 2–4 hours after tablet intake . The elimination of rivaroxaban from plasma occurs with a terminal half-life of 5–9 hours in healthy young subjects and 11–13 hours in elderly subjects .
Metabolic Pathways
Rivaroxaban, which this compound is used to quantify, undergoes metabolic degradation with approximately one-third of the dose being eliminated as unchanged active drug in the urine . It is a substrate of CYP3A4 and P-glycoprotein .
Transport and Distribution
Rivaroxaban, which this compound is used to quantify, is absorbed rapidly, with maximum plasma concentrations being reached 2–4 hours after tablet intake . It has a dual mode of excretion with the renal route accounting for one-third of the overall elimination of unchanged active drug .
Preparation Methods
Synthetic Routes and Reaction Conditions: The synthesis of Rivaroxaban-d4 involves the incorporation of deuterium atoms into the rivaroxaban molecule. This can be achieved through various methods, including catalytic hydrogen-deuterium exchange reactions. The specific synthetic route and reaction conditions may vary depending on the desired level of deuteration and the starting materials used.
Industrial Production Methods: Industrial production of this compound typically involves large-scale synthesis using optimized reaction conditions to ensure high yield and purity. The process may include steps such as deuterium gas exchange, purification, and quality control to meet the required standards for analytical use.
Chemical Reactions Analysis
Types of Reactions: Rivaroxaban-d4, like its non-deuterated counterpart, can undergo various chemical reactions, including:
Oxidation: Involves the addition of oxygen or removal of hydrogen.
Reduction: Involves the addition of hydrogen or removal of oxygen.
Substitution: Involves the replacement of one atom or group with another.
Common Reagents and Conditions:
Oxidation: Common oxidizing agents include hydrogen peroxide and potassium permanganate.
Reduction: Common reducing agents include sodium borohydride and lithium aluminum hydride.
Substitution: Common reagents include halogens and nucleophiles.
Major Products: The major products formed from these reactions depend on the specific conditions and reagents used. For example, oxidation may result in the formation of oxides, while reduction may yield reduced forms of the compound.
Comparison with Similar Compounds
Apixaban: Another direct Factor Xa inhibitor used as an anticoagulant.
Edoxaban: A direct Factor Xa inhibitor with similar applications.
Dabigatran: A direct thrombin inhibitor used as an anticoagulant.
Comparison: Rivaroxaban-d4 is unique in its use as an internal standard for analytical purposes, which distinguishes it from other anticoagulants. While apixaban, edoxaban, and dabigatran are used for similar therapeutic purposes, this compound’s deuterated form provides advantages in analytical precision and accuracy .
Properties
IUPAC Name |
5-chloro-N-[[(5S)-2-oxo-3-[2,3,5,6-tetradeuterio-4-(3-oxomorpholin-4-yl)phenyl]-1,3-oxazolidin-5-yl]methyl]thiophene-2-carboxamide | |
---|---|---|
Source | PubChem | |
URL | https://pubchem.ncbi.nlm.nih.gov | |
Description | Data deposited in or computed by PubChem | |
InChI |
InChI=1S/C19H18ClN3O5S/c20-16-6-5-15(29-16)18(25)21-9-14-10-23(19(26)28-14)13-3-1-12(2-4-13)22-7-8-27-11-17(22)24/h1-6,14H,7-11H2,(H,21,25)/t14-/m0/s1/i1D,2D,3D,4D | |
Source | PubChem | |
URL | https://pubchem.ncbi.nlm.nih.gov | |
Description | Data deposited in or computed by PubChem | |
InChI Key |
KGFYHTZWPPHNLQ-UJCPGTITSA-N | |
Source | PubChem | |
URL | https://pubchem.ncbi.nlm.nih.gov | |
Description | Data deposited in or computed by PubChem | |
Canonical SMILES |
C1COCC(=O)N1C2=CC=C(C=C2)N3CC(OC3=O)CNC(=O)C4=CC=C(S4)Cl | |
Source | PubChem | |
URL | https://pubchem.ncbi.nlm.nih.gov | |
Description | Data deposited in or computed by PubChem | |
Isomeric SMILES |
[2H]C1=C(C(=C(C(=C1N2CCOCC2=O)[2H])[2H])N3C[C@@H](OC3=O)CNC(=O)C4=CC=C(S4)Cl)[2H] | |
Source | PubChem | |
URL | https://pubchem.ncbi.nlm.nih.gov | |
Description | Data deposited in or computed by PubChem | |
Molecular Formula |
C19H18ClN3O5S | |
Source | PubChem | |
URL | https://pubchem.ncbi.nlm.nih.gov | |
Description | Data deposited in or computed by PubChem | |
DSSTOX Substance ID |
DTXSID60649494 | |
Record name | 5-Chloro-N-({(5S)-2-oxo-3-[4-(3-oxomorpholin-4-yl)(~2~H_4_)phenyl]-1,3-oxazolidin-5-yl}methyl)thiophene-2-carboxamide | |
Source | EPA DSSTox | |
URL | https://comptox.epa.gov/dashboard/DTXSID60649494 | |
Description | DSSTox provides a high quality public chemistry resource for supporting improved predictive toxicology. | |
Molecular Weight |
439.9 g/mol | |
Source | PubChem | |
URL | https://pubchem.ncbi.nlm.nih.gov | |
Description | Data deposited in or computed by PubChem | |
CAS No. |
1132681-38-9 | |
Record name | 5-Chloro-N-({(5S)-2-oxo-3-[4-(3-oxomorpholin-4-yl)(~2~H_4_)phenyl]-1,3-oxazolidin-5-yl}methyl)thiophene-2-carboxamide | |
Source | EPA DSSTox | |
URL | https://comptox.epa.gov/dashboard/DTXSID60649494 | |
Description | DSSTox provides a high quality public chemistry resource for supporting improved predictive toxicology. | |
Synthesis routes and methods
Procedure details
Retrosynthesis Analysis
AI-Powered Synthesis Planning: Our tool employs the Template_relevance Pistachio, Template_relevance Bkms_metabolic, Template_relevance Pistachio_ringbreaker, Template_relevance Reaxys, Template_relevance Reaxys_biocatalysis model, leveraging a vast database of chemical reactions to predict feasible synthetic routes.
One-Step Synthesis Focus: Specifically designed for one-step synthesis, it provides concise and direct routes for your target compounds, streamlining the synthesis process.
Accurate Predictions: Utilizing the extensive PISTACHIO, BKMS_METABOLIC, PISTACHIO_RINGBREAKER, REAXYS, REAXYS_BIOCATALYSIS database, our tool offers high-accuracy predictions, reflecting the latest in chemical research and data.
Strategy Settings
Precursor scoring | Relevance Heuristic |
---|---|
Min. plausibility | 0.01 |
Model | Template_relevance |
Template Set | Pistachio/Bkms_metabolic/Pistachio_ringbreaker/Reaxys/Reaxys_biocatalysis |
Top-N result to add to graph | 6 |
Feasible Synthetic Routes
Disclaimer and Information on In-Vitro Research Products
Please be aware that all articles and product information presented on BenchChem are intended solely for informational purposes. The products available for purchase on BenchChem are specifically designed for in-vitro studies, which are conducted outside of living organisms. In-vitro studies, derived from the Latin term "in glass," involve experiments performed in controlled laboratory settings using cells or tissues. It is important to note that these products are not categorized as medicines or drugs, and they have not received approval from the FDA for the prevention, treatment, or cure of any medical condition, ailment, or disease. We must emphasize that any form of bodily introduction of these products into humans or animals is strictly prohibited by law. It is essential to adhere to these guidelines to ensure compliance with legal and ethical standards in research and experimentation.