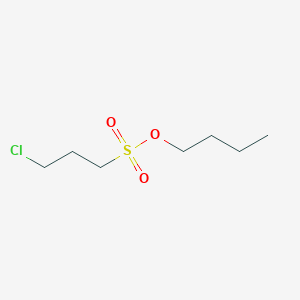
Butyl 3-chloropropylsulfonate
- Click on QUICK INQUIRY to receive a quote from our team of experts.
- With the quality product at a COMPETITIVE price, you can focus more on your research.
Overview
Description
Butyl 3-chloropropylsulfonate is an organic sulfur compound with the chemical formula C7H15ClO3S. It is a colorless to light yellow liquid with a pungent odor. This compound is soluble in organic solvents such as ethanol, dichloromethane, and methanol, but it is insoluble in water .
Preparation Methods
The preparation of Butyl 3-chloropropylsulfonate typically involves the reaction of 3-chloropropanesulfonic acid with butanol. The specific steps are as follows :
- In an appropriate reaction vessel, mix 3-chloropropanesulfonic acid and butanol in molar proportions.
- The reaction is carried out under suitable temperature and pressure conditions.
- After the reaction is complete, the product is purified and separated by distillation or other methods to obtain this compound.
Chemical Reactions Analysis
Butyl 3-chloropropylsulfonate undergoes various types of chemical reactions, including substitution reactions. Common reagents and conditions used in these reactions include nucleophiles such as amines or alcohols, which can replace the chlorine atom in the compound. The major products formed from these reactions depend on the specific nucleophile used .
Scientific Research Applications
Butyl 3-chloropropylsulfonate has several applications in scientific research, including :
Chemical Intermediate: It is commonly used as an intermediate in organic synthesis to prepare other compounds.
Drug Manufacturing: The compound can be used to synthesize certain drugs and drug intermediates.
Catalyst: It can serve as a ligand for some catalysts in various chemical reactions.
Mechanism of Action
The mechanism of action of Butyl 3-chloropropylsulfonate involves its ability to act as an electrophile due to the presence of the chlorine atom. This makes it reactive towards nucleophiles, facilitating substitution reactions. The molecular targets and pathways involved depend on the specific application and the nature of the nucleophile used .
Comparison with Similar Compounds
Butyl 3-chloropropylsulfonate can be compared with other similar compounds such as 3-chloropropanesulfonic acid butyl ester and 3-chloro-1-propanesulfonic acid butyl ester . These compounds share similar chemical structures and reactivity patterns but may differ in their specific applications and reactivity conditions. The uniqueness of this compound lies in its specific use as an intermediate in organic synthesis and its role as a ligand in catalytic reactions.
Properties
IUPAC Name |
butyl 3-chloropropane-1-sulfonate |
Source
|
---|---|---|
Source | PubChem | |
URL | https://pubchem.ncbi.nlm.nih.gov | |
Description | Data deposited in or computed by PubChem | |
InChI |
InChI=1S/C7H15ClO3S/c1-2-3-6-11-12(9,10)7-4-5-8/h2-7H2,1H3 |
Source
|
Source | PubChem | |
URL | https://pubchem.ncbi.nlm.nih.gov | |
Description | Data deposited in or computed by PubChem | |
InChI Key |
MJHBOVFGBJXYQE-UHFFFAOYSA-N |
Source
|
Source | PubChem | |
URL | https://pubchem.ncbi.nlm.nih.gov | |
Description | Data deposited in or computed by PubChem | |
Canonical SMILES |
CCCCOS(=O)(=O)CCCCl |
Source
|
Source | PubChem | |
URL | https://pubchem.ncbi.nlm.nih.gov | |
Description | Data deposited in or computed by PubChem | |
Molecular Formula |
C7H15ClO3S |
Source
|
Source | PubChem | |
URL | https://pubchem.ncbi.nlm.nih.gov | |
Description | Data deposited in or computed by PubChem | |
DSSTOX Substance ID |
DTXSID90437523 |
Source
|
Record name | Butyl 3-chloropropylsulfonate | |
Source | EPA DSSTox | |
URL | https://comptox.epa.gov/dashboard/DTXSID90437523 | |
Description | DSSTox provides a high quality public chemistry resource for supporting improved predictive toxicology. | |
Molecular Weight |
214.71 g/mol |
Source
|
Source | PubChem | |
URL | https://pubchem.ncbi.nlm.nih.gov | |
Description | Data deposited in or computed by PubChem | |
CAS No. |
146475-47-0 |
Source
|
Record name | Butyl 3-chloropropylsulfonate | |
Source | EPA DSSTox | |
URL | https://comptox.epa.gov/dashboard/DTXSID90437523 | |
Description | DSSTox provides a high quality public chemistry resource for supporting improved predictive toxicology. | |
Record name | 3-Chloro-propane-1-sulfonic acid butyl ester | |
Source | European Chemicals Agency (ECHA) | |
URL | https://echa.europa.eu/information-on-chemicals | |
Description | The European Chemicals Agency (ECHA) is an agency of the European Union which is the driving force among regulatory authorities in implementing the EU's groundbreaking chemicals legislation for the benefit of human health and the environment as well as for innovation and competitiveness. | |
Explanation | Use of the information, documents and data from the ECHA website is subject to the terms and conditions of this Legal Notice, and subject to other binding limitations provided for under applicable law, the information, documents and data made available on the ECHA website may be reproduced, distributed and/or used, totally or in part, for non-commercial purposes provided that ECHA is acknowledged as the source: "Source: European Chemicals Agency, http://echa.europa.eu/". Such acknowledgement must be included in each copy of the material. ECHA permits and encourages organisations and individuals to create links to the ECHA website under the following cumulative conditions: Links can only be made to webpages that provide a link to the Legal Notice page. | |
Disclaimer and Information on In-Vitro Research Products
Please be aware that all articles and product information presented on BenchChem are intended solely for informational purposes. The products available for purchase on BenchChem are specifically designed for in-vitro studies, which are conducted outside of living organisms. In-vitro studies, derived from the Latin term "in glass," involve experiments performed in controlled laboratory settings using cells or tissues. It is important to note that these products are not categorized as medicines or drugs, and they have not received approval from the FDA for the prevention, treatment, or cure of any medical condition, ailment, or disease. We must emphasize that any form of bodily introduction of these products into humans or animals is strictly prohibited by law. It is essential to adhere to these guidelines to ensure compliance with legal and ethical standards in research and experimentation.