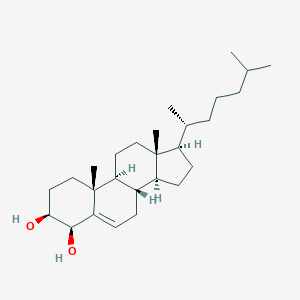
4beta-Hydroxycholesterol
Overview
Description
4beta-Hydroxycholesterol is an oxysterol, a derivative of cholesterol, where the hydrogen at the 4beta position is replaced by a hydroxy group. It is one of the major oxysterols present in human circulation and is formed by the action of the enzyme cytochrome P450 3A4. This compound plays a significant role in the regulation of cholesterol metabolism and is a ligand for liver X receptors, which are major regulators of lipid metabolism .
Mechanism of Action
Target of Action
4beta-Hydroxycholesterol (4β-HC) primarily targets the Liver X Receptor (LXR) and Cytochrome P450 3A4 (CYP3A4) . LXRs are nuclear receptors that regulate lipid metabolism , while CYP3A4 is a drug-metabolizing enzyme .
Mode of Action
4β-HC acts as an agonist for LXR , promoting the expression and activity of the master lipogenic transcription factor, SREBP1c . It also acts as a metabolite of cholesterol formed by the drug-metabolizing enzyme CYP3A4 .
Biochemical Pathways
The activation of LXR by 4β-HC leads to the induction of the lipogenic program downstream of SREBP1c . This results in the triggering of de novo lipogenesis . The PXR-4βHC-LXR pathway could link the hepatic xenobiotic exposure and the regulation of cholesterol transport in peripheral tissues .
Pharmacokinetics
4β-HC is a CYP3A metabolite of cholesterol . Its concentrations in humans are low (10–60 ng/ml), lower than many other cholesterol metabolites and far less than cholesterol itself . The stability of 4β-HC has been established for at least 12 months at −20°C in plasma samples obtained with a typical clinical workflow .
Result of Action
The action of 4β-HC leads to the repression of cholesterol influx and the induction of cholesterol efflux . It also stimulates triglyceride synthesis and lipid-droplet accumulation . In addition, it increases the expression of efflux transporters ABCA1 and ABCG1 .
Action Environment
The action of 4β-HC can be influenced by various environmental factors. For instance, the oxidation of plasma cholesterol during storage produces both 4β-HC and 4α-HC . Also, the activation of pregnane X receptor (PXR) elevates circulating 4β-HC .
Biochemical Analysis
Biochemical Properties
4beta-Hydroxycholesterol plays a significant role in biochemical reactions. It interacts with various enzymes, proteins, and other biomolecules. The compound is formed by the enzyme cytochrome P450 3A4 . It is also a ligand for liver X receptors (LXRs), which are major regulators of lipid metabolism .
Cellular Effects
This compound has profound effects on various types of cells and cellular processes. It influences cell function by impacting cell signaling pathways, gene expression, and cellular metabolism . For instance, it represses cholesterol influx and induces cholesterol efflux in human primary monocyte-derived macrophages and foam cells .
Molecular Mechanism
The molecular mechanism of this compound involves its interactions at the molecular level. It exerts its effects through binding interactions with biomolecules, enzyme inhibition or activation, and changes in gene expression . For example, it acts as a signaling molecule that represses cholesterol influx and induces efflux .
Temporal Effects in Laboratory Settings
In laboratory settings, the effects of this compound change over time. It has been established that this compound has a long half-life in plasma . The compound’s stability, degradation, and long-term effects on cellular function have been observed in in vitro or in vivo studies .
Metabolic Pathways
This compound is involved in several metabolic pathways. It is a metabolite of cholesterol formed by the enzyme cytochrome P450 3A4 . The compound also interacts with liver X receptors (LXRs), which play a crucial role in lipid metabolism .
Preparation Methods
Synthetic Routes and Reaction Conditions: 4beta-Hydroxycholesterol can be synthesized through the oxidation of cholesterol using cytochrome P450 3A4. The reaction typically involves the use of a suitable oxidizing agent under controlled conditions to ensure the selective hydroxylation at the 4beta position. The reaction conditions often include maintaining a specific temperature and pH to optimize the yield and purity of the product .
Industrial Production Methods: In industrial settings, the production of this compound involves large-scale biotransformation processes using microbial or enzymatic systems. These processes are designed to maximize the efficiency and scalability of the production. The use of recombinant microorganisms expressing cytochrome P450 3A4 is a common approach to achieve high yields of this compound .
Chemical Reactions Analysis
Types of Reactions: 4beta-Hydroxycholesterol undergoes various chemical reactions, including oxidation, reduction, and substitution. These reactions are essential for its metabolism and function within biological systems.
Common Reagents and Conditions:
Oxidation: The oxidation of this compound can be carried out using oxidizing agents such as hydrogen peroxide or molecular oxygen in the presence of catalysts.
Reduction: Reduction reactions may involve the use of reducing agents like sodium borohydride or lithium aluminum hydride.
Substitution: Substitution reactions typically involve nucleophiles such as halides or amines under specific conditions to replace the hydroxy group.
Major Products: The major products formed from these reactions include various oxysterols and other cholesterol derivatives, which play crucial roles in cellular signaling and metabolism .
Scientific Research Applications
4beta-Hydroxycholesterol has a wide range of applications in scientific research, particularly in the fields of chemistry, biology, medicine, and industry.
Chemistry: In chemistry, this compound is used as a model compound to study the mechanisms of cholesterol oxidation and the role of cytochrome P450 enzymes in these processes.
Biology: In biological research, it serves as a biomarker for the activity of cytochrome P450 3A4, providing insights into the metabolism of various drugs and endogenous compounds.
Medicine: In medicine, this compound is investigated for its potential therapeutic applications, including its role in regulating cholesterol levels and its involvement in diseases such as atherosclerosis and liver disorders.
Industry: In the industrial sector, this compound is utilized in the development of pharmaceuticals and nutraceuticals, leveraging its biological activities and regulatory functions .
Comparison with Similar Compounds
- 25-Hydroxycholesterol
- 27-Hydroxycholesterol
- 7alpha-Hydroxycholesterol
Comparison: While all these oxysterols share structural similarities and are involved in cholesterol metabolism, 4beta-Hydroxycholesterol is unique in its specific interaction with liver X receptors and its role as a biomarker for cytochrome P450 3A4 activity. This distinct interaction and regulatory function make this compound particularly valuable in both research and therapeutic contexts .
Properties
IUPAC Name |
(3S,4R,8S,9S,10R,13R,14S,17R)-10,13-dimethyl-17-[(2R)-6-methylheptan-2-yl]-2,3,4,7,8,9,11,12,14,15,16,17-dodecahydro-1H-cyclopenta[a]phenanthrene-3,4-diol | |
---|---|---|
Source | PubChem | |
URL | https://pubchem.ncbi.nlm.nih.gov | |
Description | Data deposited in or computed by PubChem | |
InChI |
InChI=1S/C27H46O2/c1-17(2)7-6-8-18(3)20-11-12-21-19-9-10-23-25(29)24(28)14-16-27(23,5)22(19)13-15-26(20,21)4/h10,17-22,24-25,28-29H,6-9,11-16H2,1-5H3/t18-,19+,20-,21+,22+,24+,25-,26-,27-/m1/s1 | |
Source | PubChem | |
URL | https://pubchem.ncbi.nlm.nih.gov | |
Description | Data deposited in or computed by PubChem | |
InChI Key |
CZDKQKOAHAICSF-JSAMMMMSSA-N | |
Source | PubChem | |
URL | https://pubchem.ncbi.nlm.nih.gov | |
Description | Data deposited in or computed by PubChem | |
Canonical SMILES |
CC(C)CCCC(C)C1CCC2C1(CCC3C2CC=C4C3(CCC(C4O)O)C)C | |
Source | PubChem | |
URL | https://pubchem.ncbi.nlm.nih.gov | |
Description | Data deposited in or computed by PubChem | |
Isomeric SMILES |
C[C@H](CCCC(C)C)[C@H]1CC[C@@H]2[C@@]1(CC[C@H]3[C@H]2CC=C4[C@@]3(CC[C@@H]([C@@H]4O)O)C)C | |
Source | PubChem | |
URL | https://pubchem.ncbi.nlm.nih.gov | |
Description | Data deposited in or computed by PubChem | |
Molecular Formula |
C27H46O2 | |
Source | PubChem | |
URL | https://pubchem.ncbi.nlm.nih.gov | |
Description | Data deposited in or computed by PubChem | |
Molecular Weight |
402.7 g/mol | |
Source | PubChem | |
URL | https://pubchem.ncbi.nlm.nih.gov | |
Description | Data deposited in or computed by PubChem | |
Physical Description |
Solid | |
Record name | 4b-Hydroxycholesterol | |
Source | Human Metabolome Database (HMDB) | |
URL | http://www.hmdb.ca/metabolites/HMDB0013643 | |
Description | The Human Metabolome Database (HMDB) is a freely available electronic database containing detailed information about small molecule metabolites found in the human body. | |
Explanation | HMDB is offered to the public as a freely available resource. Use and re-distribution of the data, in whole or in part, for commercial purposes requires explicit permission of the authors and explicit acknowledgment of the source material (HMDB) and the original publication (see the HMDB citing page). We ask that users who download significant portions of the database cite the HMDB paper in any resulting publications. | |
CAS No. |
17320-10-4 | |
Record name | 4β-Hydroxycholesterol | |
Source | CAS Common Chemistry | |
URL | https://commonchemistry.cas.org/detail?cas_rn=17320-10-4 | |
Description | CAS Common Chemistry is an open community resource for accessing chemical information. Nearly 500,000 chemical substances from CAS REGISTRY cover areas of community interest, including common and frequently regulated chemicals, and those relevant to high school and undergraduate chemistry classes. This chemical information, curated by our expert scientists, is provided in alignment with our mission as a division of the American Chemical Society. | |
Explanation | The data from CAS Common Chemistry is provided under a CC-BY-NC 4.0 license, unless otherwise stated. | |
Record name | Cholest-5-ene-3,4-diol | |
Source | ChemIDplus | |
URL | https://pubchem.ncbi.nlm.nih.gov/substance/?source=chemidplus&sourceid=0017320104 | |
Description | ChemIDplus is a free, web search system that provides access to the structure and nomenclature authority files used for the identification of chemical substances cited in National Library of Medicine (NLM) databases, including the TOXNET system. | |
Record name | 4.BETA.-HYDROXYCHOLESTEROL | |
Source | FDA Global Substance Registration System (GSRS) | |
URL | https://gsrs.ncats.nih.gov/ginas/app/beta/substances/3T705H20G7 | |
Description | The FDA Global Substance Registration System (GSRS) enables the efficient and accurate exchange of information on what substances are in regulated products. Instead of relying on names, which vary across regulatory domains, countries, and regions, the GSRS knowledge base makes it possible for substances to be defined by standardized, scientific descriptions. | |
Explanation | Unless otherwise noted, the contents of the FDA website (www.fda.gov), both text and graphics, are not copyrighted. They are in the public domain and may be republished, reprinted and otherwise used freely by anyone without the need to obtain permission from FDA. Credit to the U.S. Food and Drug Administration as the source is appreciated but not required. | |
Retrosynthesis Analysis
AI-Powered Synthesis Planning: Our tool employs the Template_relevance Pistachio, Template_relevance Bkms_metabolic, Template_relevance Pistachio_ringbreaker, Template_relevance Reaxys, Template_relevance Reaxys_biocatalysis model, leveraging a vast database of chemical reactions to predict feasible synthetic routes.
One-Step Synthesis Focus: Specifically designed for one-step synthesis, it provides concise and direct routes for your target compounds, streamlining the synthesis process.
Accurate Predictions: Utilizing the extensive PISTACHIO, BKMS_METABOLIC, PISTACHIO_RINGBREAKER, REAXYS, REAXYS_BIOCATALYSIS database, our tool offers high-accuracy predictions, reflecting the latest in chemical research and data.
Strategy Settings
Precursor scoring | Relevance Heuristic |
---|---|
Min. plausibility | 0.01 |
Model | Template_relevance |
Template Set | Pistachio/Bkms_metabolic/Pistachio_ringbreaker/Reaxys/Reaxys_biocatalysis |
Top-N result to add to graph | 6 |
Feasible Synthetic Routes
Disclaimer and Information on In-Vitro Research Products
Please be aware that all articles and product information presented on BenchChem are intended solely for informational purposes. The products available for purchase on BenchChem are specifically designed for in-vitro studies, which are conducted outside of living organisms. In-vitro studies, derived from the Latin term "in glass," involve experiments performed in controlled laboratory settings using cells or tissues. It is important to note that these products are not categorized as medicines or drugs, and they have not received approval from the FDA for the prevention, treatment, or cure of any medical condition, ailment, or disease. We must emphasize that any form of bodily introduction of these products into humans or animals is strictly prohibited by law. It is essential to adhere to these guidelines to ensure compliance with legal and ethical standards in research and experimentation.