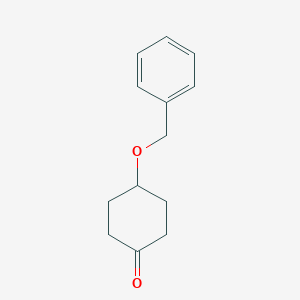
4-(Benzyloxy)cyclohexanone
Overview
Description
4-(Benzyloxy)cyclohexanone is an organic compound with the molecular formula C13H16O2. It is a colorless to light yellow liquid with a distinctive aromatic odor. This compound is soluble in organic solvents such as chloroform, dichloromethane, ethyl acetate, and methanol, but only slightly soluble in water . It is commonly used as an intermediate in organic synthesis and as a precursor for various chemical reactions.
Mechanism of Action
Target of Action
It is known that cyclohexanone derivatives are often used in the synthesis of pharmaceuticals and natural products .
Mode of Action
It is known that cyclohexanone and its derivatives can undergo various chemical reactions, including [3,3]-sigmatropic rearrangement . This rearrangement is a type of pericyclic reaction that results in the reorganization of the molecule’s structure .
Biochemical Pathways
Cyclohexanone and its derivatives are known to be involved in various chemical reactions, including [3,3]-sigmatropic rearrangement . This rearrangement can lead to the formation of new compounds with potential biological activity .
Pharmacokinetics
The compound’s lipophilicity and water solubility, which can impact its bioavailability, are noted .
Result of Action
It is known that cyclohexanone and its derivatives can undergo various chemical reactions, leading to the formation of new compounds with potential biological activity .
Preparation Methods
Synthetic Routes and Reaction Conditions: 4-(Benzyloxy)cyclohexanone can be synthesized through the condensation reaction of benzyl bromide with cyclohexanone in the presence of a base such as sodium hydride in anhydrous tetrahydrofuran (THF) at low temperatures . Another method involves the reaction of 4-hydroxycyclohexanone with benzyl chloride under basic conditions .
Industrial Production Methods: Industrial production of this compound typically involves the same synthetic routes but on a larger scale, with optimized reaction conditions to ensure high yield and purity. The use of continuous flow reactors and automated systems can enhance the efficiency and scalability of the production process .
Chemical Reactions Analysis
Types of Reactions: 4-(Benzyloxy)cyclohexanone undergoes various chemical reactions, including:
Oxidation: It can be oxidized to form this compound oxime.
Reduction: Reduction reactions can convert it to 4-(benzyloxy)cyclohexanol.
Substitution: It can undergo nucleophilic substitution reactions to form derivatives with different functional groups.
Common Reagents and Conditions:
Oxidation: Common oxidizing agents include potassium permanganate and chromium trioxide.
Reduction: Sodium borohydride or lithium aluminum hydride are typically used as reducing agents.
Substitution: Reagents such as alkyl halides or acyl chlorides can be used for substitution reactions.
Major Products Formed:
Oxidation: this compound oxime.
Reduction: 4-(Benzyloxy)cyclohexanol.
Substitution: Various substituted cyclohexanone derivatives.
Scientific Research Applications
4-(Benzyloxy)cyclohexanone has a wide range of applications in scientific research:
Comparison with Similar Compounds
4-Hydroxycyclohexanone: Similar in structure but lacks the benzyloxy group.
4-Methoxycyclohexanone: Contains a methoxy group instead of a benzyloxy group.
4-Phenylcyclohexanone: Has a phenyl group instead of a benzyloxy group.
Uniqueness: 4-(Benzyloxy)cyclohexanone is unique due to its benzyloxy group, which imparts distinct chemical and physical properties. This group enhances its solubility in organic solvents and its reactivity in various chemical reactions, making it a valuable intermediate in organic synthesis .
Properties
IUPAC Name |
4-phenylmethoxycyclohexan-1-one | |
---|---|---|
Source | PubChem | |
URL | https://pubchem.ncbi.nlm.nih.gov | |
Description | Data deposited in or computed by PubChem | |
InChI |
InChI=1S/C13H16O2/c14-12-6-8-13(9-7-12)15-10-11-4-2-1-3-5-11/h1-5,13H,6-10H2 | |
Source | PubChem | |
URL | https://pubchem.ncbi.nlm.nih.gov | |
Description | Data deposited in or computed by PubChem | |
InChI Key |
FFGVIYUANJAFJS-UHFFFAOYSA-N | |
Source | PubChem | |
URL | https://pubchem.ncbi.nlm.nih.gov | |
Description | Data deposited in or computed by PubChem | |
Canonical SMILES |
C1CC(=O)CCC1OCC2=CC=CC=C2 | |
Source | PubChem | |
URL | https://pubchem.ncbi.nlm.nih.gov | |
Description | Data deposited in or computed by PubChem | |
Molecular Formula |
C13H16O2 | |
Source | PubChem | |
URL | https://pubchem.ncbi.nlm.nih.gov | |
Description | Data deposited in or computed by PubChem | |
DSSTOX Substance ID |
DTXSID30450877 | |
Record name | 4-(Benzyloxy)cyclohexanone | |
Source | EPA DSSTox | |
URL | https://comptox.epa.gov/dashboard/DTXSID30450877 | |
Description | DSSTox provides a high quality public chemistry resource for supporting improved predictive toxicology. | |
Molecular Weight |
204.26 g/mol | |
Source | PubChem | |
URL | https://pubchem.ncbi.nlm.nih.gov | |
Description | Data deposited in or computed by PubChem | |
CAS No. |
2987-06-6 | |
Record name | 4-(Benzyloxy)cyclohexanone | |
Source | EPA DSSTox | |
URL | https://comptox.epa.gov/dashboard/DTXSID30450877 | |
Description | DSSTox provides a high quality public chemistry resource for supporting improved predictive toxicology. | |
Record name | 4-(BENZYLOXY)CYCLOHEXANONE | |
Source | European Chemicals Agency (ECHA) | |
URL | https://echa.europa.eu/information-on-chemicals | |
Description | The European Chemicals Agency (ECHA) is an agency of the European Union which is the driving force among regulatory authorities in implementing the EU's groundbreaking chemicals legislation for the benefit of human health and the environment as well as for innovation and competitiveness. | |
Explanation | Use of the information, documents and data from the ECHA website is subject to the terms and conditions of this Legal Notice, and subject to other binding limitations provided for under applicable law, the information, documents and data made available on the ECHA website may be reproduced, distributed and/or used, totally or in part, for non-commercial purposes provided that ECHA is acknowledged as the source: "Source: European Chemicals Agency, http://echa.europa.eu/". Such acknowledgement must be included in each copy of the material. ECHA permits and encourages organisations and individuals to create links to the ECHA website under the following cumulative conditions: Links can only be made to webpages that provide a link to the Legal Notice page. | |
Synthesis routes and methods I
Procedure details
Synthesis routes and methods II
Procedure details
Synthesis routes and methods III
Procedure details
Synthesis routes and methods IV
Procedure details
Retrosynthesis Analysis
AI-Powered Synthesis Planning: Our tool employs the Template_relevance Pistachio, Template_relevance Bkms_metabolic, Template_relevance Pistachio_ringbreaker, Template_relevance Reaxys, Template_relevance Reaxys_biocatalysis model, leveraging a vast database of chemical reactions to predict feasible synthetic routes.
One-Step Synthesis Focus: Specifically designed for one-step synthesis, it provides concise and direct routes for your target compounds, streamlining the synthesis process.
Accurate Predictions: Utilizing the extensive PISTACHIO, BKMS_METABOLIC, PISTACHIO_RINGBREAKER, REAXYS, REAXYS_BIOCATALYSIS database, our tool offers high-accuracy predictions, reflecting the latest in chemical research and data.
Strategy Settings
Precursor scoring | Relevance Heuristic |
---|---|
Min. plausibility | 0.01 |
Model | Template_relevance |
Template Set | Pistachio/Bkms_metabolic/Pistachio_ringbreaker/Reaxys/Reaxys_biocatalysis |
Top-N result to add to graph | 6 |
Feasible Synthetic Routes
Q1: What is the significance of the synthetic route for 4-(Benzyloxy)cyclohexanone described in the research paper?
A1: The paper "Einige Derivate und Umwandlungsprodukte des Chinits" [] outlines a method for the partial benzylation of quinitol, yielding 4-benzyloxy-cyclohexanol. This compound is then oxidized to produce this compound. This synthetic route is significant because it provides a pathway to obtain a specifically substituted cyclohexanone derivative. [] The presence of the benzyloxy group at the 4-position can be valuable for further chemical modifications and potentially influence the molecule's reactivity and properties.
Disclaimer and Information on In-Vitro Research Products
Please be aware that all articles and product information presented on BenchChem are intended solely for informational purposes. The products available for purchase on BenchChem are specifically designed for in-vitro studies, which are conducted outside of living organisms. In-vitro studies, derived from the Latin term "in glass," involve experiments performed in controlled laboratory settings using cells or tissues. It is important to note that these products are not categorized as medicines or drugs, and they have not received approval from the FDA for the prevention, treatment, or cure of any medical condition, ailment, or disease. We must emphasize that any form of bodily introduction of these products into humans or animals is strictly prohibited by law. It is essential to adhere to these guidelines to ensure compliance with legal and ethical standards in research and experimentation.