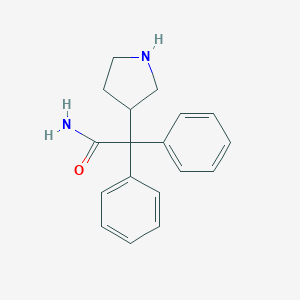
2,2-Diphenyl-2-(pyrrolidin-3-yl)acetamide
Overview
Description
2,2-Diphenyl-2-(pyrrolidin-3-yl)acetamide is a synthetic compound that belongs to the class of N-phenylpyrrolidin-2-ylacetamides. It has a molecular formula of C20H22N2O and is known for its potential therapeutic and industrial applications. This compound is characterized by the presence of a pyrrolidine ring attached to a diphenylacetamide moiety, which contributes to its unique chemical properties and biological activities.
Preparation Methods
The synthesis of 2,2-Diphenyl-2-(pyrrolidin-3-yl)acetamide can be achieved through various synthetic routes. One common method involves the reaction of diphenylacetonitrile with pyrrolidine in the presence of a suitable catalyst. The reaction conditions typically include the use of solvents such as dichloromethane or ethanol, and the reaction is carried out at room temperature or under reflux conditions .
Industrial production methods for this compound may involve large-scale synthesis using similar reaction conditions but optimized for higher yields and purity. The use of continuous flow reactors and advanced purification techniques can enhance the efficiency of the production process.
Chemical Reactions Analysis
2,2-Diphenyl-2-(pyrrolidin-3-yl)acetamide undergoes various chemical reactions, including oxidation, reduction, and substitution reactions. Common reagents used in these reactions include oxidizing agents such as potassium permanganate or hydrogen peroxide, reducing agents like sodium borohydride, and nucleophiles for substitution reactions .
Oxidation: The compound can be oxidized to form corresponding ketones or carboxylic acids.
Reduction: Reduction reactions can yield amines or alcohols.
Substitution: Nucleophilic substitution reactions can introduce various functional groups into the molecule, leading to the formation of derivatives with different properties.
Scientific Research Applications
2,2-Diphenyl-2-(pyrrolidin-3-yl)acetamide has been studied for its potential applications in various fields of scientific research. In chemistry, it is used as a building block for the synthesis of more complex molecules. In biology and medicine, it has shown promise as a therapeutic agent due to its ability to interact with specific molecular targets.
In the pharmaceutical industry, this compound is being investigated for its potential use in the treatment of neurological disorders, pain management, and as an anti-inflammatory agent. Its unique chemical structure allows it to modulate various biological pathways, making it a valuable candidate for drug development.
Mechanism of Action
The mechanism of action of 2,2-Diphenyl-2-(pyrrolidin-3-yl)acetamide involves its interaction with specific molecular targets in the body. The pyrrolidine ring and diphenylacetamide moiety enable the compound to bind to receptors and enzymes, modulating their activity. This interaction can lead to changes in cellular signaling pathways, resulting in therapeutic effects .
For example, the compound may act as an agonist or antagonist at certain neurotransmitter receptors, influencing neuronal activity and providing relief from pain or inflammation. The exact molecular targets and pathways involved can vary depending on the specific application and the biological system being studied .
Comparison with Similar Compounds
2,2-Diphenyl-2-(pyrrolidin-3-yl)acetamide can be compared with other similar compounds, such as pyrrolidine-2-one and pyrrolidine-2,5-diones. These compounds share the pyrrolidine ring structure but differ in their functional groups and overall chemical properties .
Pyrrolidine-2-one: This compound has a ketone functional group, which can influence its reactivity and biological activity.
Pyrrolidine-2,5-diones: These compounds contain two carbonyl groups, making them more reactive in certain chemical reactions.
The uniqueness of this compound lies in its diphenylacetamide moiety, which provides additional steric and electronic effects, enhancing its interaction with molecular targets and its potential therapeutic applications .
Properties
IUPAC Name |
2,2-diphenyl-2-pyrrolidin-3-ylacetamide | |
---|---|---|
Source | PubChem | |
URL | https://pubchem.ncbi.nlm.nih.gov | |
Description | Data deposited in or computed by PubChem | |
InChI |
InChI=1S/C18H20N2O/c19-17(21)18(16-11-12-20-13-16,14-7-3-1-4-8-14)15-9-5-2-6-10-15/h1-10,16,20H,11-13H2,(H2,19,21) | |
Source | PubChem | |
URL | https://pubchem.ncbi.nlm.nih.gov | |
Description | Data deposited in or computed by PubChem | |
InChI Key |
IVJSBKKYHVODFT-UHFFFAOYSA-N | |
Source | PubChem | |
URL | https://pubchem.ncbi.nlm.nih.gov | |
Description | Data deposited in or computed by PubChem | |
Canonical SMILES |
C1CNCC1C(C2=CC=CC=C2)(C3=CC=CC=C3)C(=O)N | |
Source | PubChem | |
URL | https://pubchem.ncbi.nlm.nih.gov | |
Description | Data deposited in or computed by PubChem | |
Molecular Formula |
C18H20N2O | |
Source | PubChem | |
URL | https://pubchem.ncbi.nlm.nih.gov | |
Description | Data deposited in or computed by PubChem | |
DSSTOX Substance ID |
DTXSID30545499 | |
Record name | 2,2-Diphenyl-2-(pyrrolidin-3-yl)acetamide | |
Source | EPA DSSTox | |
URL | https://comptox.epa.gov/dashboard/DTXSID30545499 | |
Description | DSSTox provides a high quality public chemistry resource for supporting improved predictive toxicology. | |
Molecular Weight |
280.4 g/mol | |
Source | PubChem | |
URL | https://pubchem.ncbi.nlm.nih.gov | |
Description | Data deposited in or computed by PubChem | |
CAS No. |
103887-32-7 | |
Record name | 2,2-Diphenyl-2-(pyrrolidin-3-yl)acetamide | |
Source | EPA DSSTox | |
URL | https://comptox.epa.gov/dashboard/DTXSID30545499 | |
Description | DSSTox provides a high quality public chemistry resource for supporting improved predictive toxicology. | |
Synthesis routes and methods I
Procedure details
Synthesis routes and methods II
Procedure details
Retrosynthesis Analysis
AI-Powered Synthesis Planning: Our tool employs the Template_relevance Pistachio, Template_relevance Bkms_metabolic, Template_relevance Pistachio_ringbreaker, Template_relevance Reaxys, Template_relevance Reaxys_biocatalysis model, leveraging a vast database of chemical reactions to predict feasible synthetic routes.
One-Step Synthesis Focus: Specifically designed for one-step synthesis, it provides concise and direct routes for your target compounds, streamlining the synthesis process.
Accurate Predictions: Utilizing the extensive PISTACHIO, BKMS_METABOLIC, PISTACHIO_RINGBREAKER, REAXYS, REAXYS_BIOCATALYSIS database, our tool offers high-accuracy predictions, reflecting the latest in chemical research and data.
Strategy Settings
Precursor scoring | Relevance Heuristic |
---|---|
Min. plausibility | 0.01 |
Model | Template_relevance |
Template Set | Pistachio/Bkms_metabolic/Pistachio_ringbreaker/Reaxys/Reaxys_biocatalysis |
Top-N result to add to graph | 6 |
Feasible Synthetic Routes
Q1: What are the identified by-products formed during the synthesis of darifenacin hydrobromide, and how were they characterized?
A1: During the development of darifenacin hydrobromide, researchers observed three by-products through HPLC analysis. These by-products, present in trace amounts (0.06-0.20%), were deemed significant enough to warrant identification and characterization due to regulatory requirements [, ]. To achieve this, the researchers undertook a comprehensive study involving synthesis and isolation of these by-products. Confirmation was achieved through co-injection with darifenacin hydrobromide, where the relative retention times (RRT) of the synthesized compounds matched those of the observed by-products [, ].
Q2: Why is the identification and characterization of by-products important in pharmaceutical development, particularly for compounds like darifenacin hydrobromide?
A2: Regulatory agencies set stringent requirements on the purity of pharmaceutical compounds like darifenacin hydrobromide. By-products, even in trace amounts exceeding 0.1%, must be identified and characterized [, ]. This is critical because:
Disclaimer and Information on In-Vitro Research Products
Please be aware that all articles and product information presented on BenchChem are intended solely for informational purposes. The products available for purchase on BenchChem are specifically designed for in-vitro studies, which are conducted outside of living organisms. In-vitro studies, derived from the Latin term "in glass," involve experiments performed in controlled laboratory settings using cells or tissues. It is important to note that these products are not categorized as medicines or drugs, and they have not received approval from the FDA for the prevention, treatment, or cure of any medical condition, ailment, or disease. We must emphasize that any form of bodily introduction of these products into humans or animals is strictly prohibited by law. It is essential to adhere to these guidelines to ensure compliance with legal and ethical standards in research and experimentation.