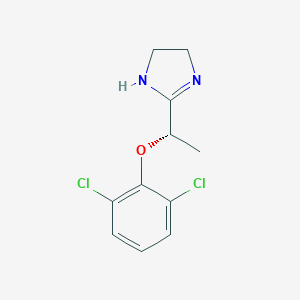
Dexlofexidine
Overview
Description
Dexlofexidine is a chemical compound known for its role as an alpha-2 adrenergic receptor agonist It is an isomer of lofexidine, which is primarily used to alleviate withdrawal symptoms in individuals discontinuing opioid use
Preparation Methods
Synthetic Routes and Reaction Conditions
The synthesis of dexlofexidine typically involves the preparation of its hydrochloride salt form. The process begins with the reaction of 2,6-dichlorophenol with ethylene oxide to form 2,6-dichlorophenoxyethanol. This intermediate is then reacted with imidazole to yield this compound. The reaction conditions often involve controlled temperatures and the use of appropriate solvents to ensure high yield and purity .
Industrial Production Methods
Industrial production of this compound follows a similar synthetic route but on a larger scale. The process involves the use of large reactors and precise control of reaction parameters to maintain consistency and quality. The final product is typically purified through crystallization or other separation techniques to achieve the desired purity levels.
Chemical Reactions Analysis
Types of Reactions
Dexlofexidine undergoes several types of chemical reactions, including:
Oxidation: this compound can be oxidized under specific conditions to form various oxidation products.
Reduction: Reduction reactions can convert this compound into its reduced forms, which may have different pharmacological properties.
Substitution: Substitution reactions involving this compound can lead to the formation of derivatives with altered chemical and biological properties.
Common Reagents and Conditions
Oxidation: Common oxidizing agents include potassium permanganate and hydrogen peroxide.
Reduction: Reducing agents such as sodium borohydride and lithium aluminum hydride are often used.
Substitution: Substitution reactions may involve reagents like halogens or other nucleophiles under controlled conditions.
Major Products Formed
The major products formed from these reactions depend on the specific reagents and conditions used. For example, oxidation may yield hydroxylated derivatives, while reduction can produce dechlorinated compounds.
Scientific Research Applications
Dexlofexidine has a wide range of scientific research applications, including:
Chemistry: It is used as a reference compound in studies involving alpha-2 adrenergic receptor agonists.
Biology: this compound is studied for its effects on cellular signaling pathways and receptor interactions.
Medicine: It is investigated for its potential use in managing withdrawal symptoms and other medical conditions.
Industry: This compound is used in the development of new pharmaceuticals and therapeutic agents
Mechanism of Action
Dexlofexidine exerts its effects by binding to alpha-2 adrenergic receptors in the central nervous system. This binding inhibits the release of norepinephrine, leading to a reduction in sympathetic nervous system activity. The result is a calming effect, which helps alleviate withdrawal symptoms and other conditions associated with overactive sympathetic outflow .
Comparison with Similar Compounds
Similar Compounds
Lofexidine: An isomer of dexlofexidine, used for similar purposes in managing opioid withdrawal symptoms.
Clonidine: Another alpha-2 adrenergic receptor agonist, commonly used for hypertension and withdrawal management.
Uniqueness
This compound is unique due to its specific isomeric form, which may result in different pharmacokinetic and pharmacodynamic properties compared to its isomers and analogs. Its reduced potency compared to levlofexidine makes it a subject of interest for further research and potential therapeutic applications .
Properties
IUPAC Name |
2-[(1S)-1-(2,6-dichlorophenoxy)ethyl]-4,5-dihydro-1H-imidazole | |
---|---|---|
Source | PubChem | |
URL | https://pubchem.ncbi.nlm.nih.gov | |
Description | Data deposited in or computed by PubChem | |
InChI |
InChI=1S/C11H12Cl2N2O/c1-7(11-14-5-6-15-11)16-10-8(12)3-2-4-9(10)13/h2-4,7H,5-6H2,1H3,(H,14,15)/t7-/m0/s1 | |
Source | PubChem | |
URL | https://pubchem.ncbi.nlm.nih.gov | |
Description | Data deposited in or computed by PubChem | |
InChI Key |
KSMAGQUYOIHWFS-ZETCQYMHSA-N | |
Source | PubChem | |
URL | https://pubchem.ncbi.nlm.nih.gov | |
Description | Data deposited in or computed by PubChem | |
Canonical SMILES |
CC(C1=NCCN1)OC2=C(C=CC=C2Cl)Cl | |
Source | PubChem | |
URL | https://pubchem.ncbi.nlm.nih.gov | |
Description | Data deposited in or computed by PubChem | |
Isomeric SMILES |
C[C@@H](C1=NCCN1)OC2=C(C=CC=C2Cl)Cl | |
Source | PubChem | |
URL | https://pubchem.ncbi.nlm.nih.gov | |
Description | Data deposited in or computed by PubChem | |
Molecular Formula |
C11H12Cl2N2O | |
Source | PubChem | |
URL | https://pubchem.ncbi.nlm.nih.gov | |
Description | Data deposited in or computed by PubChem | |
DSSTOX Substance ID |
DTXSID901024658 | |
Record name | (S)-2-(1-(2,6-Dichlorophenoxy)ethyl)-2-imidazoline | |
Source | EPA DSSTox | |
URL | https://comptox.epa.gov/dashboard/DTXSID901024658 | |
Description | DSSTox provides a high quality public chemistry resource for supporting improved predictive toxicology. | |
Molecular Weight |
259.13 g/mol | |
Source | PubChem | |
URL | https://pubchem.ncbi.nlm.nih.gov | |
Description | Data deposited in or computed by PubChem | |
CAS No. |
81447-79-2 | |
Record name | Dexlofexidine [INN] | |
Source | ChemIDplus | |
URL | https://pubchem.ncbi.nlm.nih.gov/substance/?source=chemidplus&sourceid=0081447792 | |
Description | ChemIDplus is a free, web search system that provides access to the structure and nomenclature authority files used for the identification of chemical substances cited in National Library of Medicine (NLM) databases, including the TOXNET system. | |
Record name | (S)-2-(1-(2,6-Dichlorophenoxy)ethyl)-2-imidazoline | |
Source | EPA DSSTox | |
URL | https://comptox.epa.gov/dashboard/DTXSID901024658 | |
Description | DSSTox provides a high quality public chemistry resource for supporting improved predictive toxicology. | |
Record name | DEXLOFEXIDINE | |
Source | FDA Global Substance Registration System (GSRS) | |
URL | https://gsrs.ncats.nih.gov/ginas/app/beta/substances/VXJ7Z24RN1 | |
Description | The FDA Global Substance Registration System (GSRS) enables the efficient and accurate exchange of information on what substances are in regulated products. Instead of relying on names, which vary across regulatory domains, countries, and regions, the GSRS knowledge base makes it possible for substances to be defined by standardized, scientific descriptions. | |
Explanation | Unless otherwise noted, the contents of the FDA website (www.fda.gov), both text and graphics, are not copyrighted. They are in the public domain and may be republished, reprinted and otherwise used freely by anyone without the need to obtain permission from FDA. Credit to the U.S. Food and Drug Administration as the source is appreciated but not required. | |
Synthesis routes and methods I
Procedure details
Synthesis routes and methods II
Procedure details
Synthesis routes and methods III
Procedure details
Synthesis routes and methods IV
Procedure details
Synthesis routes and methods V
Procedure details
Retrosynthesis Analysis
AI-Powered Synthesis Planning: Our tool employs the Template_relevance Pistachio, Template_relevance Bkms_metabolic, Template_relevance Pistachio_ringbreaker, Template_relevance Reaxys, Template_relevance Reaxys_biocatalysis model, leveraging a vast database of chemical reactions to predict feasible synthetic routes.
One-Step Synthesis Focus: Specifically designed for one-step synthesis, it provides concise and direct routes for your target compounds, streamlining the synthesis process.
Accurate Predictions: Utilizing the extensive PISTACHIO, BKMS_METABOLIC, PISTACHIO_RINGBREAKER, REAXYS, REAXYS_BIOCATALYSIS database, our tool offers high-accuracy predictions, reflecting the latest in chemical research and data.
Strategy Settings
Precursor scoring | Relevance Heuristic |
---|---|
Min. plausibility | 0.01 |
Model | Template_relevance |
Template Set | Pistachio/Bkms_metabolic/Pistachio_ringbreaker/Reaxys/Reaxys_biocatalysis |
Top-N result to add to graph | 6 |
Feasible Synthetic Routes
Disclaimer and Information on In-Vitro Research Products
Please be aware that all articles and product information presented on BenchChem are intended solely for informational purposes. The products available for purchase on BenchChem are specifically designed for in-vitro studies, which are conducted outside of living organisms. In-vitro studies, derived from the Latin term "in glass," involve experiments performed in controlled laboratory settings using cells or tissues. It is important to note that these products are not categorized as medicines or drugs, and they have not received approval from the FDA for the prevention, treatment, or cure of any medical condition, ailment, or disease. We must emphasize that any form of bodily introduction of these products into humans or animals is strictly prohibited by law. It is essential to adhere to these guidelines to ensure compliance with legal and ethical standards in research and experimentation.