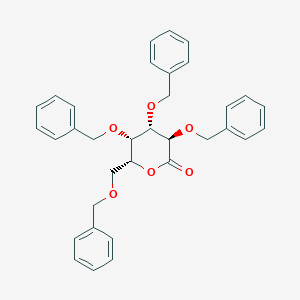
2,3,4,6-Tetra-O-benzyl-D-galactono-1,5-lactone
Overview
Description
2,3,4,6-Tetra-O-benzyl-D-galactono-1,5-lactone is a biochemical reagent primarily used in glycobiology research. It is a lactone derivative of galacturonic acid, which is a cyclic ester possessing a carbonyl group as part of the ring. This compound is significant in the study of carbohydrate chemistry, glycan formation and degradation, protein-glycan recognition, and the role of glycans in biological systems .
Mechanism of Action
Target of Action
It is used as a reactant to synthesize various glucoside containing compounds .
Mode of Action
It is known to be used in the synthesis of glucoside compounds, which are known to interact with various biological targets .
Result of Action
It is known to have significant applications within the biomedical sector, showing efficacy in combatting an array of ailments, including cancer and neurodegenerative disorders .
Preparation Methods
The synthesis of 2,3,4,6-Tetra-O-benzyl-D-galactono-1,5-lactone can be achieved through several methods, including esterification, ketalization, and lactonization. Typically, it is obtained by benzylating galactaric acid and then treating the benzylated derivative with acid
Chemical Reactions Analysis
2,3,4,6-Tetra-O-benzyl-D-galactono-1,5-lactone undergoes various chemical reactions, including oxidation, reduction, and substitution. Common reagents used in these reactions include acids, bases, and oxidizing agents. The major products formed from these reactions depend on the specific conditions and reagents used. For example, hydrolysis of the compound can yield its corresponding acid form .
Scientific Research Applications
This compound finds extensive application within the biomedical sector. It is used as a protecting group for hydroxyl groups in organic synthesis, preparation of chiral ligands for catalysis, and as a building block for the synthesis of new materials . Additionally, it has shown potential in combating ailments such as cancer and neurodegenerative disorders. Its role in glycobiology research involves studying the structure, synthesis, biology, and evolution of sugars .
Comparison with Similar Compounds
2,3,4,6-Tetra-O-benzyl-D-galactono-1,5-lactone is unique due to its specific structure and properties. Similar compounds include 2,3,4,6-Tetra-O-benzyl-D-galactose, 2,3,4,6-Tetra-O-benzyl-D-glucopyranose, and 2,3,4,6-Tetra-O-benzyl-D-gluconic acid-δ-lactone . These compounds share similar benzylated structures but differ in their specific functional groups and applications.
Properties
IUPAC Name |
(3R,4S,5S,6R)-3,4,5-tris(phenylmethoxy)-6-(phenylmethoxymethyl)oxan-2-one | |
---|---|---|
Source | PubChem | |
URL | https://pubchem.ncbi.nlm.nih.gov | |
Description | Data deposited in or computed by PubChem | |
InChI |
InChI=1S/C34H34O6/c35-34-33(39-24-29-19-11-4-12-20-29)32(38-23-28-17-9-3-10-18-28)31(37-22-27-15-7-2-8-16-27)30(40-34)25-36-21-26-13-5-1-6-14-26/h1-20,30-33H,21-25H2/t30-,31+,32+,33-/m1/s1 | |
Source | PubChem | |
URL | https://pubchem.ncbi.nlm.nih.gov | |
Description | Data deposited in or computed by PubChem | |
InChI Key |
BUBVLQDEIIUIQG-JDIHBLRXSA-N | |
Source | PubChem | |
URL | https://pubchem.ncbi.nlm.nih.gov | |
Description | Data deposited in or computed by PubChem | |
Canonical SMILES |
C1=CC=C(C=C1)COCC2C(C(C(C(=O)O2)OCC3=CC=CC=C3)OCC4=CC=CC=C4)OCC5=CC=CC=C5 | |
Source | PubChem | |
URL | https://pubchem.ncbi.nlm.nih.gov | |
Description | Data deposited in or computed by PubChem | |
Isomeric SMILES |
C1=CC=C(C=C1)COC[C@@H]2[C@@H]([C@@H]([C@H](C(=O)O2)OCC3=CC=CC=C3)OCC4=CC=CC=C4)OCC5=CC=CC=C5 | |
Source | PubChem | |
URL | https://pubchem.ncbi.nlm.nih.gov | |
Description | Data deposited in or computed by PubChem | |
Molecular Formula |
C34H34O6 | |
Source | PubChem | |
URL | https://pubchem.ncbi.nlm.nih.gov | |
Description | Data deposited in or computed by PubChem | |
DSSTOX Substance ID |
DTXSID30453681 | |
Record name | 2,3,4,6-Tetra-O-benzyl-D-galactono-1,5-lactone | |
Source | EPA DSSTox | |
URL | https://comptox.epa.gov/dashboard/DTXSID30453681 | |
Description | DSSTox provides a high quality public chemistry resource for supporting improved predictive toxicology. | |
Molecular Weight |
538.6 g/mol | |
Source | PubChem | |
URL | https://pubchem.ncbi.nlm.nih.gov | |
Description | Data deposited in or computed by PubChem | |
CAS No. |
82598-84-3 | |
Record name | 2,3,4,6-Tetra-O-benzyl-D-galactono-1,5-lactone | |
Source | EPA DSSTox | |
URL | https://comptox.epa.gov/dashboard/DTXSID30453681 | |
Description | DSSTox provides a high quality public chemistry resource for supporting improved predictive toxicology. | |
Retrosynthesis Analysis
AI-Powered Synthesis Planning: Our tool employs the Template_relevance Pistachio, Template_relevance Bkms_metabolic, Template_relevance Pistachio_ringbreaker, Template_relevance Reaxys, Template_relevance Reaxys_biocatalysis model, leveraging a vast database of chemical reactions to predict feasible synthetic routes.
One-Step Synthesis Focus: Specifically designed for one-step synthesis, it provides concise and direct routes for your target compounds, streamlining the synthesis process.
Accurate Predictions: Utilizing the extensive PISTACHIO, BKMS_METABOLIC, PISTACHIO_RINGBREAKER, REAXYS, REAXYS_BIOCATALYSIS database, our tool offers high-accuracy predictions, reflecting the latest in chemical research and data.
Strategy Settings
Precursor scoring | Relevance Heuristic |
---|---|
Min. plausibility | 0.01 |
Model | Template_relevance |
Template Set | Pistachio/Bkms_metabolic/Pistachio_ringbreaker/Reaxys/Reaxys_biocatalysis |
Top-N result to add to graph | 6 |
Feasible Synthetic Routes
Disclaimer and Information on In-Vitro Research Products
Please be aware that all articles and product information presented on BenchChem are intended solely for informational purposes. The products available for purchase on BenchChem are specifically designed for in-vitro studies, which are conducted outside of living organisms. In-vitro studies, derived from the Latin term "in glass," involve experiments performed in controlled laboratory settings using cells or tissues. It is important to note that these products are not categorized as medicines or drugs, and they have not received approval from the FDA for the prevention, treatment, or cure of any medical condition, ailment, or disease. We must emphasize that any form of bodily introduction of these products into humans or animals is strictly prohibited by law. It is essential to adhere to these guidelines to ensure compliance with legal and ethical standards in research and experimentation.