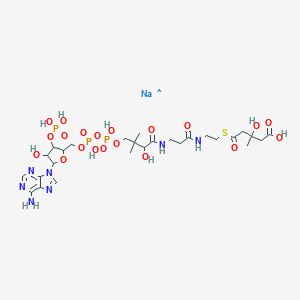
DL-3-Hydroxy-3-methylglutaryl coenzyme A sodium salt
Overview
Description
DL-3-Hydroxy-3-methylglutaryl coenzyme A sodium salt is a key intermediate in several metabolic pathways, including the biosynthesis of terpenes and ketone bodies. It is obtained from acetyl coenzyme A and acetoacetyl coenzyme A during condensation with the help of 3-hydroxy-3-methylglutaryl coenzyme A synthase . This compound is crucial in the regulation of cholesterol synthesis and is a target for statin drugs used to control cholesterol levels .
Mechanism of Action
Target of Action
DL-3-Hydroxy-3-methylglutaryl coenzyme A (HMG-CoA) sodium salt primarily targets the enzyme 3-hydroxy-3-methyl-glutaryl coenzyme A (HMG-CoA) reductase . This enzyme plays a crucial role in the biosynthesis of cholesterol , terpenes, and ketone bodies .
Mode of Action
HMG-CoA sodium salt acts as a substrate for the HMG-CoA reductase enzyme . It interacts with this enzyme to facilitate the conversion of HMG-CoA to mevalonate, a key step in the cholesterol biosynthetic pathway .
Biochemical Pathways
HMG-CoA sodium salt is involved in several metabolic pathways. The conversion of HMG-CoA to mevalonate by HMG-CoA reductase is the rate-limiting first step in the cholesterol biosynthetic pathway . Alternatively, HMG-CoA can be cleaved into acetyl-CoA and the ketone body acetoacetate in mitochondria by HMG-CoA lyase . HMG-CoA is also an intermediate in the degradation of leucine .
Pharmacokinetics
It’s known that the compound is involved in ester metabolism in vivo .
Result of Action
The action of HMG-CoA sodium salt leads to the synthesis of cholesterol, a vital component of cell membranes and precursor of steroid hormones . Inhibition of HMG-CoA can decrease cholesterol synthesis . It can also be converted to β-hydroxybutyrate in the blood .
Biochemical Analysis
Biochemical Properties
DL-3-Hydroxy-3-methylglutaryl coenzyme A sodium salt plays a crucial role in biochemical reactions. It is a substrate used to study the specificity and kinetics of the enzyme 3-hydroxyl-3-methyl-glutaryl coenzyme A (HMG-CoA) reductase . This enzyme is responsible for the conversion of HMG-CoA to mevalonate, a critical step in cholesterol and isoprenoid synthesis .
Cellular Effects
This compound has significant effects on various types of cells and cellular processes. It influences cell function by participating in the synthesis of cholesterol, a vital component of cell membranes. It also impacts cell signaling pathways and gene expression through its role in the production of isoprenoids, which are involved in protein prenylation .
Molecular Mechanism
The molecular mechanism of this compound involves its conversion to mevalonate by the enzyme HMG-CoA reductase. This reaction is the rate-limiting step in the mevalonate pathway that leads to the production of cholesterol and other isoprenoids. The product of this reaction, mevalonate, is then further processed in a series of enzymatic reactions to produce isopentenyl pyrophosphate, the basic building block for the synthesis of all isoprenoids .
Temporal Effects in Laboratory Settings
In laboratory settings, the effects of this compound can change over time. The stability of this compound and its degradation products can influence the outcomes of biochemical reactions. Long-term effects on cellular function have been observed in in vitro or in vivo studies, particularly in relation to cholesterol synthesis and regulation .
Dosage Effects in Animal Models
The effects of this compound can vary with different dosages in animal models. High doses of this compound can lead to increased production of cholesterol and other isoprenoids, which can have various effects on cellular function and overall organism health .
Metabolic Pathways
This compound is involved in the mevalonate pathway, a crucial metabolic pathway for the production of cholesterol and isoprenoids. This pathway involves a series of enzymatic reactions that convert acetyl-CoA into isopentenyl pyrophosphate .
Transport and Distribution
This compound is transported and distributed within cells and tissues as part of its role in the mevalonate pathway. The transport of this compound and its intermediates is critical for the proper functioning of this pathway .
Subcellular Localization
This compound and its related intermediates are localized in the cytosol, where the mevalonate pathway takes place. The enzymes involved in this pathway are also primarily located in the cytosol .
Preparation Methods
Synthetic Routes and Reaction Conditions
DL-3-Hydroxy-3-methylglutaryl coenzyme A sodium salt is synthesized from acetyl coenzyme A and acetoacetyl coenzyme A through a condensation reaction catalyzed by 3-hydroxy-3-methylglutaryl coenzyme A synthase . The reaction typically occurs in the presence of a suitable buffer and at a controlled temperature to ensure optimal enzyme activity.
Industrial Production Methods
Industrial production of this compound involves large-scale fermentation processes using genetically engineered microorganisms that overexpress 3-hydroxy-3-methylglutaryl coenzyme A synthase. The fermentation broth is then subjected to purification steps, including filtration, chromatography, and crystallization, to obtain the pure compound .
Chemical Reactions Analysis
Types of Reactions
DL-3-Hydroxy-3-methylglutaryl coenzyme A sodium salt undergoes several types of chemical reactions, including:
Oxidation: Conversion to mevalonate by 3-hydroxy-3-methylglutaryl coenzyme A reductase.
Cleavage: Cleavage into acetyl coenzyme A and acetoacetate by 3-hydroxy-3-methylglutaryl coenzyme A lyase.
Common Reagents and Conditions
Oxidation: Requires 3-hydroxy-3-methylglutaryl coenzyme A reductase and NADPH as a reducing agent.
Cleavage: Catalyzed by 3-hydroxy-3-methylglutaryl coenzyme A lyase in the presence of suitable cofactors.
Major Products
Oxidation: Mevalonate.
Cleavage: Acetyl coenzyme A and acetoacetate.
Scientific Research Applications
DL-3-Hydroxy-3-methylglutaryl coenzyme A sodium salt has numerous scientific research applications:
Comparison with Similar Compounds
DL-3-Hydroxy-3-methylglutaryl coenzyme A sodium salt is unique due to its role as a key intermediate in cholesterol biosynthesis. Similar compounds include:
Mevalonate: A product of the reduction of this compound.
Acetoacetyl coenzyme A: A precursor in the synthesis of this compound.
Acetyl coenzyme A: Another precursor in the synthesis of this compound.
This compound stands out due to its regulatory role in cholesterol biosynthesis and its significance as a target for statin drugs .
Properties
InChI |
InChI=1S/C27H44N7O20P3S.Na/c1-26(2,21(40)24(41)30-5-4-15(35)29-6-7-58-17(38)9-27(3,42)8-16(36)37)11-51-57(48,49)54-56(46,47)50-10-14-20(53-55(43,44)45)19(39)25(52-14)34-13-33-18-22(28)31-12-32-23(18)34;/h12-14,19-21,25,39-40,42H,4-11H2,1-3H3,(H,29,35)(H,30,41)(H,36,37)(H,46,47)(H,48,49)(H2,28,31,32)(H2,43,44,45); | |
---|---|---|
Source | PubChem | |
URL | https://pubchem.ncbi.nlm.nih.gov | |
Description | Data deposited in or computed by PubChem | |
InChI Key |
KMNHIBBYYDCBIJ-UHFFFAOYSA-N | |
Source | PubChem | |
URL | https://pubchem.ncbi.nlm.nih.gov | |
Description | Data deposited in or computed by PubChem | |
Canonical SMILES |
CC(C)(COP(=O)(O)OP(=O)(O)OCC1C(C(C(O1)N2C=NC3=C(N=CN=C32)N)O)OP(=O)(O)O)C(C(=O)NCCC(=O)NCCSC(=O)CC(C)(CC(=O)O)O)O.[Na] | |
Source | PubChem | |
URL | https://pubchem.ncbi.nlm.nih.gov | |
Description | Data deposited in or computed by PubChem | |
Molecular Formula |
C27H44N7NaO20P3S | |
Source | PubChem | |
URL | https://pubchem.ncbi.nlm.nih.gov | |
Description | Data deposited in or computed by PubChem | |
DSSTOX Substance ID |
DTXSID30585178 | |
Record name | DL-3-Hydroxy-3-methylglutaryl coenzyme A sodium salt | |
Source | EPA DSSTox | |
URL | https://comptox.epa.gov/dashboard/DTXSID30585178 | |
Description | DSSTox provides a high quality public chemistry resource for supporting improved predictive toxicology. | |
Molecular Weight |
934.7 g/mol | |
Source | PubChem | |
URL | https://pubchem.ncbi.nlm.nih.gov | |
Description | Data deposited in or computed by PubChem | |
CAS No. |
103476-21-7 | |
Record name | DL-3-Hydroxy-3-methylglutaryl coenzyme A sodium salt | |
Source | EPA DSSTox | |
URL | https://comptox.epa.gov/dashboard/DTXSID30585178 | |
Description | DSSTox provides a high quality public chemistry resource for supporting improved predictive toxicology. | |
Retrosynthesis Analysis
AI-Powered Synthesis Planning: Our tool employs the Template_relevance Pistachio, Template_relevance Bkms_metabolic, Template_relevance Pistachio_ringbreaker, Template_relevance Reaxys, Template_relevance Reaxys_biocatalysis model, leveraging a vast database of chemical reactions to predict feasible synthetic routes.
One-Step Synthesis Focus: Specifically designed for one-step synthesis, it provides concise and direct routes for your target compounds, streamlining the synthesis process.
Accurate Predictions: Utilizing the extensive PISTACHIO, BKMS_METABOLIC, PISTACHIO_RINGBREAKER, REAXYS, REAXYS_BIOCATALYSIS database, our tool offers high-accuracy predictions, reflecting the latest in chemical research and data.
Strategy Settings
Precursor scoring | Relevance Heuristic |
---|---|
Min. plausibility | 0.01 |
Model | Template_relevance |
Template Set | Pistachio/Bkms_metabolic/Pistachio_ringbreaker/Reaxys/Reaxys_biocatalysis |
Top-N result to add to graph | 6 |
Feasible Synthetic Routes
Disclaimer and Information on In-Vitro Research Products
Please be aware that all articles and product information presented on BenchChem are intended solely for informational purposes. The products available for purchase on BenchChem are specifically designed for in-vitro studies, which are conducted outside of living organisms. In-vitro studies, derived from the Latin term "in glass," involve experiments performed in controlled laboratory settings using cells or tissues. It is important to note that these products are not categorized as medicines or drugs, and they have not received approval from the FDA for the prevention, treatment, or cure of any medical condition, ailment, or disease. We must emphasize that any form of bodily introduction of these products into humans or animals is strictly prohibited by law. It is essential to adhere to these guidelines to ensure compliance with legal and ethical standards in research and experimentation.