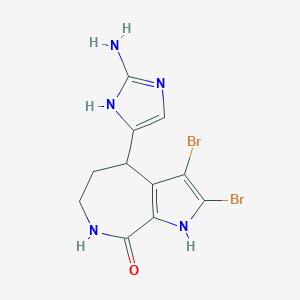
(+/-)-Hymenin
Overview
Description
(+/-)-Hymenin is a naturally occurring compound found in various plant species. It is known for its diverse biological activities and potential therapeutic applications. The compound is a member of the flavonoid family, which is characterized by a common phenylbenzopyrone structure. Flavonoids are widely recognized for their antioxidant, anti-inflammatory, and anticancer properties.
Preparation Methods
Synthetic Routes and Reaction Conditions
The synthesis of (+/-)-Hymenin typically involves the cyclization of appropriate precursors under specific conditions. One common method is the use of chalcone intermediates, which undergo cyclization in the presence of acidic or basic catalysts. The reaction conditions often include elevated temperatures and the use of solvents such as ethanol or methanol.
Industrial Production Methods
Industrial production of this compound can be achieved through the extraction from natural sources or via chemical synthesis. Extraction involves the use of solvents to isolate the compound from plant materials, followed by purification steps such as chromatography. Chemical synthesis, on the other hand, involves the stepwise construction of the flavonoid structure using commercially available starting materials.
Chemical Reactions Analysis
Types of Reactions
(+/-)-Hymenin undergoes various chemical reactions, including:
Oxidation: The compound can be oxidized to form quinones or other oxidized derivatives.
Reduction: Reduction reactions can convert this compound to its corresponding dihydroflavonoid.
Substitution: Electrophilic and nucleophilic substitution reactions can introduce different functional groups onto the flavonoid core.
Common Reagents and Conditions
Oxidation: Common oxidizing agents include potassium permanganate and hydrogen peroxide.
Reduction: Reducing agents such as sodium borohydride or lithium aluminum hydride are often used.
Substitution: Reagents like halogens, alkyl halides, and nucleophiles are employed under various conditions, including acidic or basic environments.
Major Products
Scientific Research Applications
(+/-)-Hymenin has a wide range of scientific research applications:
Chemistry: It is used as a model compound for studying flavonoid chemistry and reactivity.
Biology: The compound is investigated for its role in plant defense mechanisms and its interactions with other biomolecules.
Medicine: this compound is studied for its potential therapeutic effects, including antioxidant, anti-inflammatory, and anticancer activities.
Industry: The compound is used in the development of natural health products, dietary supplements, and cosmetics.
Mechanism of Action
The mechanism of action of (+/-)-Hymenin involves its interaction with various molecular targets and pathways. The compound exerts its effects primarily through its antioxidant activity, scavenging free radicals and reducing oxidative stress. Additionally, this compound modulates signaling pathways involved in inflammation and cell proliferation, contributing to its anti-inflammatory and anticancer properties.
Comparison with Similar Compounds
(+/-)-Hymenin can be compared with other flavonoids such as quercetin, kaempferol, and myricetin. While all these compounds share a common flavonoid structure, this compound is unique in its specific substitution pattern and biological activities. For instance, quercetin is known for its strong antioxidant properties, while kaempferol exhibits significant anti-inflammatory effects. Myricetin, on the other hand, is recognized for its anticancer potential. The unique combination of properties in this compound makes it a valuable compound for various applications.
List of Similar Compounds
- Quercetin
- Kaempferol
- Myricetin
Properties
IUPAC Name |
4-(2-amino-1H-imidazol-5-yl)-2,3-dibromo-4,5,6,7-tetrahydro-1H-pyrrolo[2,3-c]azepin-8-one | |
---|---|---|
Source | PubChem | |
URL | https://pubchem.ncbi.nlm.nih.gov | |
Description | Data deposited in or computed by PubChem | |
InChI |
InChI=1S/C11H11Br2N5O/c12-7-6-4(5-3-16-11(14)17-5)1-2-15-10(19)8(6)18-9(7)13/h3-4,18H,1-2H2,(H,15,19)(H3,14,16,17) | |
Source | PubChem | |
URL | https://pubchem.ncbi.nlm.nih.gov | |
Description | Data deposited in or computed by PubChem | |
InChI Key |
MRMGABUTBNWSLA-UHFFFAOYSA-N | |
Source | PubChem | |
URL | https://pubchem.ncbi.nlm.nih.gov | |
Description | Data deposited in or computed by PubChem | |
Canonical SMILES |
C1CNC(=O)C2=C(C1C3=CN=C(N3)N)C(=C(N2)Br)Br | |
Source | PubChem | |
URL | https://pubchem.ncbi.nlm.nih.gov | |
Description | Data deposited in or computed by PubChem | |
Molecular Formula |
C11H11Br2N5O | |
Source | PubChem | |
URL | https://pubchem.ncbi.nlm.nih.gov | |
Description | Data deposited in or computed by PubChem | |
DSSTOX Substance ID |
DTXSID30433004 | |
Record name | (+/-)-Hymenin | |
Source | EPA DSSTox | |
URL | https://comptox.epa.gov/dashboard/DTXSID30433004 | |
Description | DSSTox provides a high quality public chemistry resource for supporting improved predictive toxicology. | |
Molecular Weight |
389.05 g/mol | |
Source | PubChem | |
URL | https://pubchem.ncbi.nlm.nih.gov | |
Description | Data deposited in or computed by PubChem | |
CAS No. |
154569-13-8 | |
Record name | (+/-)-Hymenin | |
Source | EPA DSSTox | |
URL | https://comptox.epa.gov/dashboard/DTXSID30433004 | |
Description | DSSTox provides a high quality public chemistry resource for supporting improved predictive toxicology. | |
Retrosynthesis Analysis
AI-Powered Synthesis Planning: Our tool employs the Template_relevance Pistachio, Template_relevance Bkms_metabolic, Template_relevance Pistachio_ringbreaker, Template_relevance Reaxys, Template_relevance Reaxys_biocatalysis model, leveraging a vast database of chemical reactions to predict feasible synthetic routes.
One-Step Synthesis Focus: Specifically designed for one-step synthesis, it provides concise and direct routes for your target compounds, streamlining the synthesis process.
Accurate Predictions: Utilizing the extensive PISTACHIO, BKMS_METABOLIC, PISTACHIO_RINGBREAKER, REAXYS, REAXYS_BIOCATALYSIS database, our tool offers high-accuracy predictions, reflecting the latest in chemical research and data.
Strategy Settings
Precursor scoring | Relevance Heuristic |
---|---|
Min. plausibility | 0.01 |
Model | Template_relevance |
Template Set | Pistachio/Bkms_metabolic/Pistachio_ringbreaker/Reaxys/Reaxys_biocatalysis |
Top-N result to add to graph | 6 |
Feasible Synthetic Routes
Q1: What is the molecular formula and weight of (+/-)-hymenin?
A1: this compound has the molecular formula C15H20O2 and a molecular weight of 232.32 g/mol. [, ]
Q2: What spectroscopic techniques are used to characterize this compound?
A2: Researchers commonly employ NMR (1H NMR and 13C NMR), IR, and mass spectrometry to elucidate the structure and confirm the identity of this compound. [, ]
Q3: What are the primary biological activities reported for this compound?
A3: Studies have demonstrated that this compound exhibits various biological activities, including cytotoxic, anti-inflammatory, antibacterial, antifungal, and α-adrenoceptor blocking properties. [, , , , , , ]
Q4: How does this compound exert its cytotoxic effects?
A4: Research suggests that this compound induces apoptosis in cancer cells. This programmed cell death is characterized by specific morphological changes, including the translocation of phosphatidylserine to the outer cell membrane and the formation of apoptotic bodies. [, ]
Q5: What is the role of the α-methylene-γ-lactone moiety in this compound's activity?
A5: The α-methylene-γ-lactone moiety, a common structural feature in sesquiterpene lactones, appears to be crucial for the cytotoxic and antiulcerogenic activities of this compound. [, ]
Q6: Does this compound exhibit selectivity towards specific enzymes?
A6: Yes, this compound demonstrates selective inhibition towards cyclooxygenase-1 (COX-1) compared to COX-2. This selectivity might contribute to its potential anti-inflammatory effects with reduced gastrointestinal side effects compared to non-selective COX inhibitors. []
Q7: How does this compound impact the Nrf2-ARE pathway?
A7: Research indicates that this compound can act as an Nrf2 inducer. This activation of the Nrf2-ARE pathway enhances the expression of antioxidant enzymes, contributing to its neuroprotective effects against oxidative stress. []
Q8: How do structural modifications of this compound influence its biological activity?
A8: Modifications to the this compound structure can significantly impact its activity. For instance, the presence of a hydroxyl group at the C-1 position enhances cytotoxic activity, while reduction of the double bond at C-2 reduces activity against specific cancer cell lines. []
Q9: Is there evidence of cross-reactivity between this compound and other sesquiterpene lactones?
A9: While this compound shares structural similarities with other sesquiterpene lactones, studies in guinea pigs indicate a relatively high immunological specificity for this compound. This suggests limited cross-reactivity with related compounds like parthenin. []
Q10: What are the natural sources of this compound?
A10: this compound has been isolated from various plant species, including Ambrosia maritima and Parthenium hysterophorus, as well as marine sponges like Hymeniacidon sp and Stylissa massa. [, , , , ]
Q11: Have there been successful attempts to synthesize this compound?
A11: Yes, several total syntheses of (±)-hymenin have been reported, employing various strategies like gold-catalyzed intramolecular alkyne hydroarylation and functionalization of the cyclopentane ring. [, , , ]
Disclaimer and Information on In-Vitro Research Products
Please be aware that all articles and product information presented on BenchChem are intended solely for informational purposes. The products available for purchase on BenchChem are specifically designed for in-vitro studies, which are conducted outside of living organisms. In-vitro studies, derived from the Latin term "in glass," involve experiments performed in controlled laboratory settings using cells or tissues. It is important to note that these products are not categorized as medicines or drugs, and they have not received approval from the FDA for the prevention, treatment, or cure of any medical condition, ailment, or disease. We must emphasize that any form of bodily introduction of these products into humans or animals is strictly prohibited by law. It is essential to adhere to these guidelines to ensure compliance with legal and ethical standards in research and experimentation.