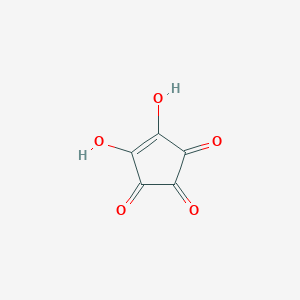
Croconic acid
Overview
Description
Croconic acid, also known as 4,5-dihydroxycyclopent-4-ene-1,2,3-trione, is a chemical compound with the formula C5H2O5. It features a cyclopentene backbone with two hydroxyl groups adjacent to the double bond and three ketone groups on the remaining carbon atoms. This compound is known for its yellow crystalline form and its sensitivity to light. This compound is soluble in water and ethanol and decomposes at temperatures above 212°C .
Mechanism of Action
Target of Action
Croconic acid, also known as 4,5-dihydroxycyclopentenetrione, is a chemical compound with the formula C5H2O5 . The primary targets of this compound are the hydrogen-bonded protons in its molecular structure . These protons play a crucial role in the compound’s ferroelectric properties .
Mode of Action
The mode of action of this compound involves the movement of protons parallel and perpendicular to the polar axis, and the consequent electronic rearrangement . This movement is believed to induce the observed polarization in this compound . The compound’s interaction with its targets results in changes in the frequencies of the well-defined doublet in inelastic neutron scattering spectra associated with out-of-plane motions of hydrogen-bonded protons .
Biochemical Pathways
The biochemical pathways affected by this compound involve the transfer of protons between molecular units . This proton transfer is a characteristic feature of organic ferroelectrics such as this compound . The downstream effects of these pathways include the weakening of bonding motifs and enhancement of proton motions .
Result of Action
The result of this compound’s action is the generation of a spontaneous, switchable electric polarization . This is due to proton transfer between adjacent molecules in each pleated sheet, rather than molecular rotation . This compound is known for its high spontaneous polarization, which can be robust up to 400 K .
Action Environment
The action, efficacy, and stability of this compound can be influenced by environmental factors such as temperature . For instance, a detailed study of the thermal behavior of atomic motions in this compound revealed that the frequencies of the well-defined doublet decrease monotonically with temperature, indicating a weakening of these bonding motifs and enhancement of proton motions . This suggests that the compound’s ferroelectric properties can be enhanced at higher temperatures .
Biochemical Analysis
Biochemical Properties
Croconic acid is acidic and loses the protons from the hydroxyl groups . The resulting anions, hydrogencroconate C5HO−5 and croconate C5O2−5, are quite stable . The croconate ion, in particular, is aromatic and symmetric, as the double bond and the negative charges become delocalized over the five CO units . The lithium, sodium, and potassium croconates crystallize from water as dihydrates .
Molecular Mechanism
The molecular mechanism of this compound involves proton tautomerism . This process is crucial for the description of ferroelectricity in this compound in terms of its Γ-point phonon modes . The potential energy curve along the minimum energy path is sensitive to the order of proton transfer .
Temporal Effects in Laboratory Settings
This compound has demonstrated ferroelectricity at room temperature in nanometer-thin quasi 2D films . The polarization hysteresis loop measurements indicate that the application of an electric field can coherently align the molecular polarities in crystalline this compound .
Subcellular Localization
In the solid state, this compound has a peculiar structure consisting of pleated strips, each “page” of the strip being a planar ring of 4 molecules of C5O5H2 held together by hydrogen bonds . This structure could potentially influence its subcellular localization.
Preparation Methods
Synthetic Routes and Reaction Conditions: Croconic acid can be synthesized through the oxidation of rhodizonic acid. The process involves the use of hydrochloric acid to crystallize this compound from an aqueous solution. The reaction conditions typically include maintaining a controlled temperature and pH to ensure the proper formation of the desired product .
Industrial Production Methods: In industrial settings, this compound is produced by dissolving raw materials in water to ensure fluidity and facilitate the reaction. The process involves the careful control of reaction parameters to optimize yield and purity .
Chemical Reactions Analysis
Types of Reactions: Croconic acid undergoes various chemical reactions, including oxidation, reduction, and substitution.
Common Reagents and Conditions:
Oxidation: this compound can be oxidized using strong oxidizing agents such as potassium permanganate or hydrogen peroxide.
Reduction: Reduction reactions typically involve the use of reducing agents like sodium borohydride.
Substitution: Substitution reactions often occur in the presence of nucleophiles, leading to the formation of ethers and esters.
Major Products: The major products formed from these reactions include croconate salts, ethers such as dimethyl croconate, and other derivatives .
Scientific Research Applications
Croconic acid has a wide range of scientific research applications:
Chemistry: It is used as a building block for the synthesis of various organic compounds and dyes.
Biology: this compound derivatives are employed as dyes for biological research purposes.
Medicine: Research is ongoing to explore its potential therapeutic applications.
Industry: Its ferroelectric properties make it suitable for use in electronic devices, sensors, and actuators
Comparison with Similar Compounds
Deltic Acid: 2,3-dihydroxycycloprop-2-ene-1-one
Squaric Acid: 3,4-dihydroxycyclobut-3-ene-1,2-dione
Rhodizonic Acid: 5,6-dihydroxycyclohex-5-ene-1,2,3,4-tetrone.
Croconic acid stands out due to its large polarization, organic nature, and potential for non-toxic and lead-free device applications .
Properties
IUPAC Name |
4,5-dihydroxycyclopent-4-ene-1,2,3-trione | |
---|---|---|
Source | PubChem | |
URL | https://pubchem.ncbi.nlm.nih.gov | |
Description | Data deposited in or computed by PubChem | |
InChI |
InChI=1S/C5H2O5/c6-1-2(7)4(9)5(10)3(1)8/h6-7H | |
Source | PubChem | |
URL | https://pubchem.ncbi.nlm.nih.gov | |
Description | Data deposited in or computed by PubChem | |
InChI Key |
RBSLJAJQOVYTRQ-UHFFFAOYSA-N | |
Source | PubChem | |
URL | https://pubchem.ncbi.nlm.nih.gov | |
Description | Data deposited in or computed by PubChem | |
Canonical SMILES |
C1(=C(C(=O)C(=O)C1=O)O)O | |
Source | PubChem | |
URL | https://pubchem.ncbi.nlm.nih.gov | |
Description | Data deposited in or computed by PubChem | |
Molecular Formula |
C5H2O5 | |
Source | PubChem | |
URL | https://pubchem.ncbi.nlm.nih.gov | |
Description | Data deposited in or computed by PubChem | |
DSSTOX Substance ID |
DTXSID20878763 | |
Record name | Croconic acid | |
Source | EPA DSSTox | |
URL | https://comptox.epa.gov/dashboard/DTXSID20878763 | |
Description | DSSTox provides a high quality public chemistry resource for supporting improved predictive toxicology. | |
Molecular Weight |
142.07 g/mol | |
Source | PubChem | |
URL | https://pubchem.ncbi.nlm.nih.gov | |
Description | Data deposited in or computed by PubChem | |
CAS No. |
488-86-8 | |
Record name | Croconic acid | |
Source | CAS Common Chemistry | |
URL | https://commonchemistry.cas.org/detail?cas_rn=488-86-8 | |
Description | CAS Common Chemistry is an open community resource for accessing chemical information. Nearly 500,000 chemical substances from CAS REGISTRY cover areas of community interest, including common and frequently regulated chemicals, and those relevant to high school and undergraduate chemistry classes. This chemical information, curated by our expert scientists, is provided in alignment with our mission as a division of the American Chemical Society. | |
Explanation | The data from CAS Common Chemistry is provided under a CC-BY-NC 4.0 license, unless otherwise stated. | |
Record name | Croconic acid | |
Source | ChemIDplus | |
URL | https://pubchem.ncbi.nlm.nih.gov/substance/?source=chemidplus&sourceid=0000488868 | |
Description | ChemIDplus is a free, web search system that provides access to the structure and nomenclature authority files used for the identification of chemical substances cited in National Library of Medicine (NLM) databases, including the TOXNET system. | |
Record name | Croconic acid | |
Source | EPA DSSTox | |
URL | https://comptox.epa.gov/dashboard/DTXSID20878763 | |
Description | DSSTox provides a high quality public chemistry resource for supporting improved predictive toxicology. | |
Record name | Croconic Acid | |
Source | European Chemicals Agency (ECHA) | |
URL | https://echa.europa.eu/information-on-chemicals | |
Description | The European Chemicals Agency (ECHA) is an agency of the European Union which is the driving force among regulatory authorities in implementing the EU's groundbreaking chemicals legislation for the benefit of human health and the environment as well as for innovation and competitiveness. | |
Explanation | Use of the information, documents and data from the ECHA website is subject to the terms and conditions of this Legal Notice, and subject to other binding limitations provided for under applicable law, the information, documents and data made available on the ECHA website may be reproduced, distributed and/or used, totally or in part, for non-commercial purposes provided that ECHA is acknowledged as the source: "Source: European Chemicals Agency, http://echa.europa.eu/". Such acknowledgement must be included in each copy of the material. ECHA permits and encourages organisations and individuals to create links to the ECHA website under the following cumulative conditions: Links can only be made to webpages that provide a link to the Legal Notice page. | |
Record name | CROCONIC ACID | |
Source | FDA Global Substance Registration System (GSRS) | |
URL | https://gsrs.ncats.nih.gov/ginas/app/beta/substances/6HGY35UXAA | |
Description | The FDA Global Substance Registration System (GSRS) enables the efficient and accurate exchange of information on what substances are in regulated products. Instead of relying on names, which vary across regulatory domains, countries, and regions, the GSRS knowledge base makes it possible for substances to be defined by standardized, scientific descriptions. | |
Explanation | Unless otherwise noted, the contents of the FDA website (www.fda.gov), both text and graphics, are not copyrighted. They are in the public domain and may be republished, reprinted and otherwise used freely by anyone without the need to obtain permission from FDA. Credit to the U.S. Food and Drug Administration as the source is appreciated but not required. | |
Retrosynthesis Analysis
AI-Powered Synthesis Planning: Our tool employs the Template_relevance Pistachio, Template_relevance Bkms_metabolic, Template_relevance Pistachio_ringbreaker, Template_relevance Reaxys, Template_relevance Reaxys_biocatalysis model, leveraging a vast database of chemical reactions to predict feasible synthetic routes.
One-Step Synthesis Focus: Specifically designed for one-step synthesis, it provides concise and direct routes for your target compounds, streamlining the synthesis process.
Accurate Predictions: Utilizing the extensive PISTACHIO, BKMS_METABOLIC, PISTACHIO_RINGBREAKER, REAXYS, REAXYS_BIOCATALYSIS database, our tool offers high-accuracy predictions, reflecting the latest in chemical research and data.
Strategy Settings
Precursor scoring | Relevance Heuristic |
---|---|
Min. plausibility | 0.01 |
Model | Template_relevance |
Template Set | Pistachio/Bkms_metabolic/Pistachio_ringbreaker/Reaxys/Reaxys_biocatalysis |
Top-N result to add to graph | 6 |
Feasible Synthetic Routes
Disclaimer and Information on In-Vitro Research Products
Please be aware that all articles and product information presented on BenchChem are intended solely for informational purposes. The products available for purchase on BenchChem are specifically designed for in-vitro studies, which are conducted outside of living organisms. In-vitro studies, derived from the Latin term "in glass," involve experiments performed in controlled laboratory settings using cells or tissues. It is important to note that these products are not categorized as medicines or drugs, and they have not received approval from the FDA for the prevention, treatment, or cure of any medical condition, ailment, or disease. We must emphasize that any form of bodily introduction of these products into humans or animals is strictly prohibited by law. It is essential to adhere to these guidelines to ensure compliance with legal and ethical standards in research and experimentation.