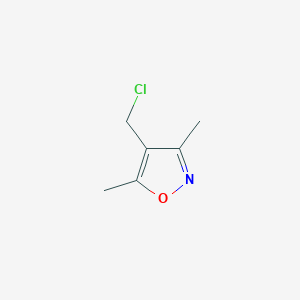
4-(Chloromethyl)-3,5-dimethylisoxazole
Overview
Description
4-(Chloromethyl)-3,5-dimethylisoxazole (CM-DMI) is an organic compound that is widely used in scientific research. It is a colorless, crystalline solid that is insoluble in water and has a molecular weight of 150.58 g/mol. CM-DMI is a versatile compound that is used in various laboratory experiments and has several biochemical and physiological effects.
Scientific Research Applications
Synthesis of New Derivatives
The compound can be used in the synthesis of new derivatives of other compounds. For instance, it has been used in the synthesis of new calix4arene derivatives . These derivatives have shown promising results in various fields, including medicinal chemistry .
Cytotoxic Activity
The compound and its derivatives have been evaluated for their cytotoxic activity. They have shown to inhibit the proliferation of different human cancer cells, including DLD-1, A549, HEPG2, and PC-3 cells in a dose-dependent manner . This suggests potential applications in cancer research and treatment.
Chloromethylation of Aromatic Compounds
“4-(Chloromethyl)-3,5-dimethylisoxazole” can be used in the chloromethylation of aromatic compounds . Chloromethyl substituted aromatic compounds are promising key intermediates because of their easy transformation to a variety of fine or special chemicals, polymers, and pharmaceuticals .
Catalyst in Chemical Reactions
The compound can also act as a catalyst in certain chemical reactions. For example, it has been used as a catalyst in the chloromethylation of benzene with dimethoxymethane and chlorosulfonic acid .
Production of Fine or Special Chemicals
As mentioned earlier, the chloromethyl substituted aromatic compounds, which can be produced using “4-(Chloromethyl)-3,5-dimethylisoxazole”, can be easily transformed into a variety of fine or special chemicals . This makes it valuable in the chemical industry.
Production of Polymers
The compound can also be used in the production of polymers. The chloromethyl substituted aromatic compounds, which can be produced using “4-(Chloromethyl)-3,5-dimethylisoxazole”, can be used in the synthesis of various polymers .
Safety and Hazards
According to the safety data sheet, 4-(Chloromethyl)-3,5-dimethylisoxazole is considered hazardous. It may cause skin irritation, serious eye irritation, and respiratory irritation. It is recommended to avoid breathing dust/fume/gas/mist/vapors/spray and to use only outdoors or in a well-ventilated area .
Mechanism of Action
Target of Action
Similar compounds have been used in suzuki–miyaura coupling reactions, which are widely applied transition metal-catalyzed carbon–carbon bond-forming reactions .
Mode of Action
In the context of suzuki–miyaura coupling reactions, the compound may interact with its targets through a process involving oxidative addition and transmetalation . Oxidative addition occurs with formally electrophilic organic groups, whereby palladium becomes oxidized through its donation of electrons to form a new Pd–C bond. Transmetalation occurs with formally nucleophilic organic groups, which are transferred from boron to palladium .
Biochemical Pathways
It’s worth noting that the compound could potentially be involved in various biochemical transformations due to its reactivity in carbon–carbon bond-forming reactions .
Pharmacokinetics
Similar compounds have been analyzed using reverse phase (rp) hplc methods, which could potentially provide insights into the compound’s bioavailability .
Result of Action
The compound’s potential role in suzuki–miyaura coupling reactions suggests it could facilitate the formation of new carbon–carbon bonds, thereby enabling the synthesis of complex organic molecules .
Action Environment
The action, efficacy, and stability of 4-(Chloromethyl)-3,5-dimethylisoxazole can be influenced by various environmental factors. For instance, the compound’s reactivity in Suzuki–Miyaura coupling reactions can be affected by the presence of a suitable catalyst and the reaction conditions . Additionally, safety data sheets suggest that the compound should be handled with care to avoid risks associated with inhalation, skin contact, and eye contact .
properties
IUPAC Name |
4-(chloromethyl)-3,5-dimethyl-1,2-oxazole | |
---|---|---|
Source | PubChem | |
URL | https://pubchem.ncbi.nlm.nih.gov | |
Description | Data deposited in or computed by PubChem | |
InChI |
InChI=1S/C6H8ClNO/c1-4-6(3-7)5(2)9-8-4/h3H2,1-2H3 | |
Source | PubChem | |
URL | https://pubchem.ncbi.nlm.nih.gov | |
Description | Data deposited in or computed by PubChem | |
InChI Key |
NIFAUKBQIAURIM-UHFFFAOYSA-N | |
Source | PubChem | |
URL | https://pubchem.ncbi.nlm.nih.gov | |
Description | Data deposited in or computed by PubChem | |
Canonical SMILES |
CC1=C(C(=NO1)C)CCl | |
Source | PubChem | |
URL | https://pubchem.ncbi.nlm.nih.gov | |
Description | Data deposited in or computed by PubChem | |
Molecular Formula |
C6H8ClNO | |
Source | PubChem | |
URL | https://pubchem.ncbi.nlm.nih.gov | |
Description | Data deposited in or computed by PubChem | |
DSSTOX Substance ID |
DTXSID0066531 | |
Record name | Isoxazole, 4-(chloromethyl)-3,5-dimethyl- | |
Source | EPA DSSTox | |
URL | https://comptox.epa.gov/dashboard/DTXSID0066531 | |
Description | DSSTox provides a high quality public chemistry resource for supporting improved predictive toxicology. | |
Molecular Weight |
145.59 g/mol | |
Source | PubChem | |
URL | https://pubchem.ncbi.nlm.nih.gov | |
Description | Data deposited in or computed by PubChem | |
CAS RN |
19788-37-5 | |
Record name | 4-(Chloromethyl)-3,5-dimethylisoxazole | |
Source | CAS Common Chemistry | |
URL | https://commonchemistry.cas.org/detail?cas_rn=19788-37-5 | |
Description | CAS Common Chemistry is an open community resource for accessing chemical information. Nearly 500,000 chemical substances from CAS REGISTRY cover areas of community interest, including common and frequently regulated chemicals, and those relevant to high school and undergraduate chemistry classes. This chemical information, curated by our expert scientists, is provided in alignment with our mission as a division of the American Chemical Society. | |
Explanation | The data from CAS Common Chemistry is provided under a CC-BY-NC 4.0 license, unless otherwise stated. | |
Record name | Isoxazole, 4-(chloromethyl)-3,5-dimethyl- | |
Source | ChemIDplus | |
URL | https://pubchem.ncbi.nlm.nih.gov/substance/?source=chemidplus&sourceid=0019788375 | |
Description | ChemIDplus is a free, web search system that provides access to the structure and nomenclature authority files used for the identification of chemical substances cited in National Library of Medicine (NLM) databases, including the TOXNET system. | |
Record name | Isoxazole, 4-(chloromethyl)-3,5-dimethyl- | |
Source | EPA Chemicals under the TSCA | |
URL | https://www.epa.gov/chemicals-under-tsca | |
Description | EPA Chemicals under the Toxic Substances Control Act (TSCA) collection contains information on chemicals and their regulations under TSCA, including non-confidential content from the TSCA Chemical Substance Inventory and Chemical Data Reporting. | |
Record name | Isoxazole, 4-(chloromethyl)-3,5-dimethyl- | |
Source | EPA DSSTox | |
URL | https://comptox.epa.gov/dashboard/DTXSID0066531 | |
Description | DSSTox provides a high quality public chemistry resource for supporting improved predictive toxicology. | |
Record name | 4-(chloromethyl)-3,5-dimethylisoxazole | |
Source | European Chemicals Agency (ECHA) | |
URL | https://echa.europa.eu/substance-information/-/substanceinfo/100.039.360 | |
Description | The European Chemicals Agency (ECHA) is an agency of the European Union which is the driving force among regulatory authorities in implementing the EU's groundbreaking chemicals legislation for the benefit of human health and the environment as well as for innovation and competitiveness. | |
Explanation | Use of the information, documents and data from the ECHA website is subject to the terms and conditions of this Legal Notice, and subject to other binding limitations provided for under applicable law, the information, documents and data made available on the ECHA website may be reproduced, distributed and/or used, totally or in part, for non-commercial purposes provided that ECHA is acknowledged as the source: "Source: European Chemicals Agency, http://echa.europa.eu/". Such acknowledgement must be included in each copy of the material. ECHA permits and encourages organisations and individuals to create links to the ECHA website under the following cumulative conditions: Links can only be made to webpages that provide a link to the Legal Notice page. | |
Retrosynthesis Analysis
AI-Powered Synthesis Planning: Our tool employs the Template_relevance Pistachio, Template_relevance Bkms_metabolic, Template_relevance Pistachio_ringbreaker, Template_relevance Reaxys, Template_relevance Reaxys_biocatalysis model, leveraging a vast database of chemical reactions to predict feasible synthetic routes.
One-Step Synthesis Focus: Specifically designed for one-step synthesis, it provides concise and direct routes for your target compounds, streamlining the synthesis process.
Accurate Predictions: Utilizing the extensive PISTACHIO, BKMS_METABOLIC, PISTACHIO_RINGBREAKER, REAXYS, REAXYS_BIOCATALYSIS database, our tool offers high-accuracy predictions, reflecting the latest in chemical research and data.
Strategy Settings
Precursor scoring | Relevance Heuristic |
---|---|
Min. plausibility | 0.01 |
Model | Template_relevance |
Template Set | Pistachio/Bkms_metabolic/Pistachio_ringbreaker/Reaxys/Reaxys_biocatalysis |
Top-N result to add to graph | 6 |
Feasible Synthetic Routes
Q & A
Q1: What are the key structural characteristics of 4-(Chloromethyl)-3,5-dimethylisoxazole and how have they been determined?
A1: 4-(Chloromethyl)-3,5-dimethylisoxazole is an organic compound with the molecular formula C6H8ClNO and a molecular weight of 145.59 g/mol []. Its structure has been elucidated using various spectroscopic techniques. Key findings include:
Q2: How is 4-(Chloromethyl)-3,5-dimethylisoxazole used in organic synthesis?
A2: 4-(Chloromethyl)-3,5-dimethylisoxazole is a valuable reagent in organic synthesis, particularly for constructing cyclohexenone rings []. This structural motif is found in various natural products, including steroids, terpenes, and alkaloids []. The compound's reactivity stems from the presence of the chloromethyl group, which can participate in various nucleophilic substitution reactions, enabling the formation of new carbon-carbon bonds.
Q3: Have there been any computational studies on 4-(Chloromethyl)-3,5-dimethylisoxazole and its parent compound, 3,5-dimethylisoxazole?
A3: Yes, a Density Functional Theory (DFT) study investigated the structural and electronic properties of both 3,5-dimethylisoxazole (DMI) and 4-(Chloromethyl)-3,5-dimethylisoxazole (CDMI) []. The study utilized the B3LYP/6-31+G(d,p) basis set within the Gaussian 09 software package []. Key findings include:
Disclaimer and Information on In-Vitro Research Products
Please be aware that all articles and product information presented on BenchChem are intended solely for informational purposes. The products available for purchase on BenchChem are specifically designed for in-vitro studies, which are conducted outside of living organisms. In-vitro studies, derived from the Latin term "in glass," involve experiments performed in controlled laboratory settings using cells or tissues. It is important to note that these products are not categorized as medicines or drugs, and they have not received approval from the FDA for the prevention, treatment, or cure of any medical condition, ailment, or disease. We must emphasize that any form of bodily introduction of these products into humans or animals is strictly prohibited by law. It is essential to adhere to these guidelines to ensure compliance with legal and ethical standards in research and experimentation.