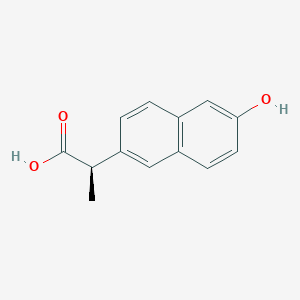
(R)-O-Desmethyl Naproxen
Overview
Description
(R)-O-Desmethyl Naproxen is a non-steroidal anti-inflammatory drug (NSAID) with a wide range of applications in the medical field. It is considered a prodrug, which means it is metabolized in the body to form the active drug, naproxen. Naproxen is a non-selective inhibitor of cyclooxygenase (COX) enzymes, which are responsible for the synthesis of prostaglandins, important mediators of inflammation. As an NSAID, this compound is a potent analgesic and anti-inflammatory agent, and has been used to treat various inflammatory conditions such as rheumatoid arthritis and osteoarthritis. It has also been studied for its potential benefits in treating certain types of cancer.
Scientific Research Applications
Solubility and Physicochemical Properties
Research has focused on enhancing the solubility of Naproxen by exploring its behavior in various solvents, including deep eutectic solvents (DES). The solubility of Naproxen increases with the concentration of DES and temperature, indicating strong interactions between Naproxen and DES. This finding could improve drug formulation and delivery methods for more efficient therapeutic effects (Mokhtarpour et al., 2019).
Environmental Impact and Degradation
Studies have also examined the environmental impact of Naproxen, including its stability and removal in wastewater treatment processes. An advanced wastewater treatment plant utilizing ultra-filtration, activated charcoal, and reverse osmosis showed efficiency in removing Naproxen, suggesting the potential for improving water purification technologies (Qurie et al., 2014). Additionally, research on the degradation of Naproxen by various chemical processes has provided insights into potential methods for reducing its presence in the environment, thereby mitigating its impact on ecosystems (Arany et al., 2013).
Mechanism of Action
Target of Action
®-O-Desmethyl Naproxen, a derivative of Naproxen, primarily targets the Cyclooxygenase (COX) enzymes . These enzymes, specifically COX-1 and COX-2, play a crucial role in the synthesis of prostaglandins, which are key mediators of inflammation and pain .
Mode of Action
®-O-Desmethyl Naproxen acts as a non-selective COX inhibitor . It works by reversibly inhibiting both COX-1 and COX-2 enzymes . This inhibition reduces the production of prostaglandins, thereby alleviating inflammation and pain .
Biochemical Pathways
The primary biochemical pathway affected by ®-O-Desmethyl Naproxen is the prostaglandin synthesis pathway . By inhibiting the COX enzymes, it disrupts the conversion of arachidonic acid to prostaglandins . This results in a decrease in prostaglandin levels, leading to reduced inflammation and pain .
Pharmacokinetics
The pharmacokinetics of ®-O-Desmethyl Naproxen is similar to that of Naproxen. Naproxen is well-absorbed orally, with a bioavailability of 95% . It binds extensively to plasma albumin, and its plasma concentration is linearly proportional to the dose for oral doses up to 500 mg . It is metabolized in the liver to 6-O-desmethylnaproxen, which is then excreted in the urine . The elimination half-life of Naproxen is approximately 12-17 hours .
Result of Action
The primary result of ®-O-Desmethyl Naproxen’s action is the reduction of inflammation and pain . By inhibiting the production of prostaglandins, it effectively manages acute pain as well as pain related to rheumatic diseases .
Action Environment
Naproxen has been detected in various types of water, including drinking water and groundwater . Although the concentrations are low, long-term exposure to Naproxen can have adverse effects on non-target organisms, especially when mixed with other drugs . The biological decomposition of Naproxen is performed by fungi, algae, and bacteria . The only well-described pathway for its complete degradation is the degradation of naproxen by bacillus thuringiensis b1(2015b) .
Safety and Hazards
®-Naproxen has several safety considerations. It is harmful if swallowed, causes skin irritation, serious eye irritation, may cause respiratory irritation, and is suspected of damaging fertility or the unborn child . It is advised to avoid contact with skin and eyes, avoid formation of dust and aerosols, and provide appropriate exhaust ventilation at places where dust is formed .
Future Directions
Biochemical Analysis
Biochemical Properties
®-O-Desmethyl Naproxen, similar to Naproxen, is likely to interact with various enzymes, proteins, and other biomolecules. Naproxen is known to inhibit the cyclooxygenase enzymes (COX-1 and COX-2), which are involved in the synthesis of prostaglandins, chemicals produced by the body that cause inflammation .
Cellular Effects
The cellular effects of ®-O-Desmethyl Naproxen are expected to be similar to those of Naproxen. Naproxen has been shown to have effects on various types of cells and cellular processes. For instance, it has been reported to inhibit TRPM7 channels in T lymphocytes . In human fibroblasts, Naproxen photosensitization induced by UVA led to a drastic reduction of cell viability .
Molecular Mechanism
The molecular mechanism of action of ®-O-Desmethyl Naproxen is likely to be similar to that of Naproxen. Naproxen works by reversibly inhibiting both the COX-1 and COX-2 enzymes as a non-selective coxib . This inhibition reduces the production of prostaglandins, thereby exerting its anti-inflammatory action .
Temporal Effects in Laboratory Settings
Studies on Naproxen have shown that it has a half-life of 12–17 hours in adults . This suggests that the effects of ®-O-Desmethyl Naproxen may also persist for a significant duration.
Dosage Effects in Animal Models
The dosage effects of ®-O-Desmethyl Naproxen in animal models have not been specifically studied. Studies on Naproxen have shown that it has analgesic and anti-inflammatory effects in various animal models .
Metabolic Pathways
Naproxen is known to be metabolized by the liver to inactive metabolites . It’s plausible that ®-O-Desmethyl Naproxen undergoes similar metabolic processes.
Transport and Distribution
Naproxen is known to bind extensively to plasma albumin, suggesting that it may be distributed throughout the body via the bloodstream .
Subcellular Localization
The subcellular localization of ®-O-Desmethyl Naproxen is not well-documented. Given its likely similarity to Naproxen, it may be expected to localize in areas where its target enzymes, COX-1 and COX-2, are found. These enzymes are typically found in the endoplasmic reticulum and nuclear envelope .
properties
IUPAC Name |
(2R)-2-(6-hydroxynaphthalen-2-yl)propanoic acid | |
---|---|---|
Source | PubChem | |
URL | https://pubchem.ncbi.nlm.nih.gov | |
Description | Data deposited in or computed by PubChem | |
InChI |
InChI=1S/C13H12O3/c1-8(13(15)16)9-2-3-11-7-12(14)5-4-10(11)6-9/h2-8,14H,1H3,(H,15,16)/t8-/m1/s1 | |
Source | PubChem | |
URL | https://pubchem.ncbi.nlm.nih.gov | |
Description | Data deposited in or computed by PubChem | |
InChI Key |
XWJUDDGELKXYNO-MRVPVSSYSA-N | |
Source | PubChem | |
URL | https://pubchem.ncbi.nlm.nih.gov | |
Description | Data deposited in or computed by PubChem | |
Canonical SMILES |
CC(C1=CC2=C(C=C1)C=C(C=C2)O)C(=O)O | |
Source | PubChem | |
URL | https://pubchem.ncbi.nlm.nih.gov | |
Description | Data deposited in or computed by PubChem | |
Isomeric SMILES |
C[C@H](C1=CC2=C(C=C1)C=C(C=C2)O)C(=O)O | |
Source | PubChem | |
URL | https://pubchem.ncbi.nlm.nih.gov | |
Description | Data deposited in or computed by PubChem | |
Molecular Formula |
C13H12O3 | |
Source | PubChem | |
URL | https://pubchem.ncbi.nlm.nih.gov | |
Description | Data deposited in or computed by PubChem | |
Molecular Weight |
216.23 g/mol | |
Source | PubChem | |
URL | https://pubchem.ncbi.nlm.nih.gov | |
Description | Data deposited in or computed by PubChem | |
Synthesis routes and methods
Procedure details
Disclaimer and Information on In-Vitro Research Products
Please be aware that all articles and product information presented on BenchChem are intended solely for informational purposes. The products available for purchase on BenchChem are specifically designed for in-vitro studies, which are conducted outside of living organisms. In-vitro studies, derived from the Latin term "in glass," involve experiments performed in controlled laboratory settings using cells or tissues. It is important to note that these products are not categorized as medicines or drugs, and they have not received approval from the FDA for the prevention, treatment, or cure of any medical condition, ailment, or disease. We must emphasize that any form of bodily introduction of these products into humans or animals is strictly prohibited by law. It is essential to adhere to these guidelines to ensure compliance with legal and ethical standards in research and experimentation.