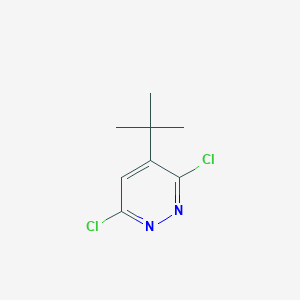
4-tert-Butyl-3,6-dichloropyridazine
Overview
Description
Synthesis Analysis
The synthesis of compounds related to 4-tert-Butyl-3,6-dichloropyridazine often involves multi-step reactions starting from simple precursors like tert-butylhydrazine, dichloroacrylic acid, and tert-butylphenyl methanethiol. A notable example includes the synthesis of 2-tert-butyl-5-(4-tert-butylbenzylthio)-4-chloropyridazin-3(2H)-one, which demonstrates the potential routes for synthesizing tert-butyl and dichloropyridazine derivatives through carefully designed synthetic pathways (Liang-zhong Xu et al., 2006).
Molecular Structure Analysis
The crystal structure analysis of similar compounds provides insight into the molecular geometry, inter- and intramolecular interactions, and stabilization mechanisms of 4-tert-Butyl-3,6-dichloropyridazine derivatives. For instance, studies on related compounds highlight orthorhombic crystal systems and detailed hydrogen bonding interactions, which are crucial for understanding the structural aspects of such molecules (Zou Xia, 2001).
Chemical Reactions and Properties
Chemical reactions involving 4-tert-Butyl-3,6-dichloropyridazine derivatives vary widely, including reactions with hydrazines to form hydrazones, pyrazolines, or pyridazinones, depending on the reaction conditions. Such reactivity patterns offer a glimpse into the versatile chemistry of tert-butyl and dichloropyridazine-containing compounds, which can be manipulated for the synthesis of a wide range of derivatives (Javier Figueroa et al., 2006).
Scientific Research Applications
Synthesis of Complex Molecules
4-tert-Butyl-3,6-dichloropyridazine has been utilized in the synthesis of complex molecular structures, such as dinuclear complexes and pyrazolo[3,4-c]pyridazines derivatives, which show promising applications in water oxidation and catalysis. For instance, Zong and Thummel (2005) developed a series of well-organized dinuclear complexes that demonstrate significant potential in oxygen evolution reactions, a critical process for water oxidation (Zong & Thummel, 2005). Additionally, Wunderlich and Knochel (2008) reported on the efficient mono- and bis-functionalization of 3,6-dichloropyridazine, leading to highly substituted pyrazolo[3,4-c]pyridazines derivatives, underscoring its versatility in organic synthesis (Wunderlich & Knochel, 2008).
Development of New Materials
The research on 4-tert-Butyl-3,6-dichloropyridazine extends to the development of new materials, such as light-colored fluorinated polyimides, which exhibit excellent thermal stability and electrical properties. Lu et al. (2014) synthesized a series of poly(pyridine–imide)s with tert-butyl substituents, demonstrating good solubility and thermal stability, making them suitable for advanced electronic applications (Lu et al., 2014).
Catalytic Applications
Moreover, 4-tert-Butyl-3,6-dichloropyridazine has found applications in catalysis, particularly in the synthesis of copper complexes for oxidation reactions. Manzur et al. (2000) prepared binuclear copper(II) complexes that catalyze the oxidation of 3,5-di-tert-butylcatechol, a reaction pertinent to environmental chemistry and the pharmaceutical industry (Manzur et al., 2000).
Safety and Hazards
The safety information for 4-tert-Butyl-3,6-dichloropyridazine includes several hazard statements: H302-H315-H319-H332-H335 . This indicates that the compound may be harmful if swallowed, may cause skin irritation, may cause serious eye irritation, may be harmful if inhaled, and may cause respiratory irritation . Precautionary measures include avoiding breathing dust/fume/gas/mist/vapors/spray, wearing protective gloves/protective clothing/eye protection/face protection, and rinsing cautiously with water for several minutes in case of contact with eyes .
properties
IUPAC Name |
4-tert-butyl-3,6-dichloropyridazine | |
---|---|---|
Source | PubChem | |
URL | https://pubchem.ncbi.nlm.nih.gov | |
Description | Data deposited in or computed by PubChem | |
InChI |
InChI=1S/C8H10Cl2N2/c1-8(2,3)5-4-6(9)11-12-7(5)10/h4H,1-3H3 | |
Source | PubChem | |
URL | https://pubchem.ncbi.nlm.nih.gov | |
Description | Data deposited in or computed by PubChem | |
InChI Key |
DTUZXHRABOWYAE-UHFFFAOYSA-N | |
Source | PubChem | |
URL | https://pubchem.ncbi.nlm.nih.gov | |
Description | Data deposited in or computed by PubChem | |
Canonical SMILES |
CC(C)(C)C1=CC(=NN=C1Cl)Cl | |
Source | PubChem | |
URL | https://pubchem.ncbi.nlm.nih.gov | |
Description | Data deposited in or computed by PubChem | |
Molecular Formula |
C8H10Cl2N2 | |
Source | PubChem | |
URL | https://pubchem.ncbi.nlm.nih.gov | |
Description | Data deposited in or computed by PubChem | |
DSSTOX Substance ID |
DTXSID40945486 | |
Record name | 4-tert-Butyl-3,6-dichloropyridazine | |
Source | EPA DSSTox | |
URL | https://comptox.epa.gov/dashboard/DTXSID40945486 | |
Description | DSSTox provides a high quality public chemistry resource for supporting improved predictive toxicology. | |
Molecular Weight |
205.08 g/mol | |
Source | PubChem | |
URL | https://pubchem.ncbi.nlm.nih.gov | |
Description | Data deposited in or computed by PubChem | |
Product Name |
4-tert-Butyl-3,6-dichloropyridazine | |
CAS RN |
22808-29-3 | |
Record name | 4-tert-Butyl-3,6-dichloropyridazine | |
Source | CAS Common Chemistry | |
URL | https://commonchemistry.cas.org/detail?cas_rn=22808-29-3 | |
Description | CAS Common Chemistry is an open community resource for accessing chemical information. Nearly 500,000 chemical substances from CAS REGISTRY cover areas of community interest, including common and frequently regulated chemicals, and those relevant to high school and undergraduate chemistry classes. This chemical information, curated by our expert scientists, is provided in alignment with our mission as a division of the American Chemical Society. | |
Explanation | The data from CAS Common Chemistry is provided under a CC-BY-NC 4.0 license, unless otherwise stated. | |
Record name | Pyridazine, 3,6-dichloro-4-(1,1-dimethylethyl)- | |
Source | ChemIDplus | |
URL | https://pubchem.ncbi.nlm.nih.gov/substance/?source=chemidplus&sourceid=0022808293 | |
Description | ChemIDplus is a free, web search system that provides access to the structure and nomenclature authority files used for the identification of chemical substances cited in National Library of Medicine (NLM) databases, including the TOXNET system. | |
Record name | 4-tert-Butyl-3,6-dichloropyridazine | |
Source | EPA DSSTox | |
URL | https://comptox.epa.gov/dashboard/DTXSID40945486 | |
Description | DSSTox provides a high quality public chemistry resource for supporting improved predictive toxicology. | |
Record name | 4-tert-butyl-3,6-dichloropyridazine | |
Source | European Chemicals Agency (ECHA) | |
URL | https://echa.europa.eu/information-on-chemicals | |
Description | The European Chemicals Agency (ECHA) is an agency of the European Union which is the driving force among regulatory authorities in implementing the EU's groundbreaking chemicals legislation for the benefit of human health and the environment as well as for innovation and competitiveness. | |
Explanation | Use of the information, documents and data from the ECHA website is subject to the terms and conditions of this Legal Notice, and subject to other binding limitations provided for under applicable law, the information, documents and data made available on the ECHA website may be reproduced, distributed and/or used, totally or in part, for non-commercial purposes provided that ECHA is acknowledged as the source: "Source: European Chemicals Agency, http://echa.europa.eu/". Such acknowledgement must be included in each copy of the material. ECHA permits and encourages organisations and individuals to create links to the ECHA website under the following cumulative conditions: Links can only be made to webpages that provide a link to the Legal Notice page. | |
Synthesis routes and methods I
Procedure details
Synthesis routes and methods II
Procedure details
Disclaimer and Information on In-Vitro Research Products
Please be aware that all articles and product information presented on BenchChem are intended solely for informational purposes. The products available for purchase on BenchChem are specifically designed for in-vitro studies, which are conducted outside of living organisms. In-vitro studies, derived from the Latin term "in glass," involve experiments performed in controlled laboratory settings using cells or tissues. It is important to note that these products are not categorized as medicines or drugs, and they have not received approval from the FDA for the prevention, treatment, or cure of any medical condition, ailment, or disease. We must emphasize that any form of bodily introduction of these products into humans or animals is strictly prohibited by law. It is essential to adhere to these guidelines to ensure compliance with legal and ethical standards in research and experimentation.