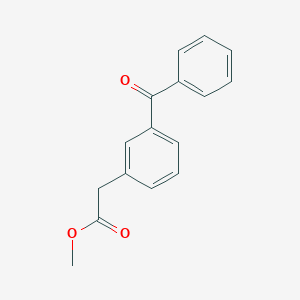
Desmethyl Ketoprofen Methyl Ester
Overview
Description
Desmethyl Ketoprofen Methyl Ester is an organic compound with the molecular formula C16H14O3. It is an ester derived from the reaction between 3-benzoylbenzoic acid and methanol. This compound is known for its applications in various fields, including chemistry, biology, and industry.
Mechanism of Action
Target of Action
Desmethyl Ketoprofen Methyl Ester, also known as Methyl 2-(3-benzoylphenyl)acetate, Methyl (3-Benzoylphenyl)acetate, or methyl m-benzoyl-phenyl-acetate, primarily targets the Cyclooxygenase-1 (COX-1) enzyme . COX-1 is a rate-limiting enzyme in the synthesis of pro-inflammatory prostanoids from arachidonic acid .
Mode of Action
The compound’s mode of action is believed to be through the inhibition of COX-1 . This inhibition results in a decrease in the synthesis of pro-inflammatory prostanoids from arachidonic acid . The compound’s interaction with its target leads to a reduction in inflammation and pain, which is characteristic of nonsteroidal anti-inflammatory drugs (NSAIDs) .
Biochemical Pathways
The primary biochemical pathway affected by this compound is the arachidonic acid pathway . By inhibiting COX-1, the compound reduces the conversion of arachidonic acid into pro-inflammatory prostanoids . This action can lead to downstream effects such as reduced inflammation and pain.
Pharmacokinetics
The compound is expected to undergo extensive biotransformation to inactive glucuroconjugated metabolites .
Result of Action
The primary result of the action of this compound is a reduction in inflammation and pain . By inhibiting COX-1, the compound reduces the production of pro-inflammatory prostanoids, leading to a decrease in inflammation . This makes it a potentially effective treatment for conditions characterized by inflammation and pain.
Biochemical Analysis
Biochemical Properties
It is known that Ketoprofen, the parent drug, inhibits the enzyme cyclooxygenase (COX), which is involved in the synthesis of pro-inflammatory prostanoids from arachidonic acid . Therefore, it is plausible that Desmethyl Ketoprofen Methyl Ester may interact with similar enzymes and proteins.
Cellular Effects
It is known that Ketoprofen, the parent drug, can influence cell function by modulating cell signaling pathways, gene expression, and cellular metabolism . It is possible that this compound may have similar effects on cells.
Molecular Mechanism
It is known that Ketoprofen, the parent drug, exerts its effects at the molecular level by inhibiting the enzyme COX, leading to a decrease in the production of pro-inflammatory prostanoids . It is possible that this compound may have a similar mechanism of action.
Metabolic Pathways
It is known that Ketoprofen, the parent drug, is metabolized in the liver through phase I and phase II metabolic reactions . It is possible that this compound may be involved in similar metabolic pathways.
Transport and Distribution
It is known that Ketoprofen, the parent drug, can cross the blood-brain barrier . It is possible that this compound may have similar transport and distribution characteristics.
Preparation Methods
Synthetic Routes and Reaction Conditions
Desmethyl Ketoprofen Methyl Ester can be synthesized through the esterification of 3-benzoylbenzoic acid with methanol in the presence of an acid catalyst, such as sulfuric acid. The reaction typically involves heating the mixture under reflux conditions to facilitate the formation of the ester. The reaction can be represented as follows:
3-Benzoylbenzoic acid+MethanolH2SO4Methyl (3-Benzoylphenyl)acetate+Water
Industrial Production Methods
In an industrial setting, the production of methyl (3-benzoylphenyl)acetate can be optimized using microwave-assisted esterification. This method enhances the reaction efficiency and reduces the reaction time compared to conventional methods. The process involves the use of microwave irradiation, which provides uniform heating and accelerates the reaction rate.
Chemical Reactions Analysis
Types of Reactions
Desmethyl Ketoprofen Methyl Ester undergoes various chemical reactions, including:
Hydrolysis: The ester can be hydrolyzed under acidic or basic conditions to yield 3-benzoylbenzoic acid and methanol.
Reduction: Reduction of the ester can produce the corresponding alcohol.
Substitution: The ester group can be substituted with other functional groups through nucleophilic acyl substitution reactions.
Common Reagents and Conditions
Hydrolysis: Acidic hydrolysis involves the use of hydrochloric acid, while basic hydrolysis uses sodium hydroxide.
Reduction: Lithium aluminum hydride (LiAlH4) is commonly used as a reducing agent.
Substitution: Grignard reagents or organolithium compounds can be used for nucleophilic acyl substitution.
Major Products Formed
Hydrolysis: 3-Benzoylbenzoic acid and methanol.
Reduction: The corresponding alcohol.
Substitution: Various substituted esters or amides, depending on the nucleophile used.
Scientific Research Applications
Desmethyl Ketoprofen Methyl Ester has several applications in scientific research:
Chemistry: It is used as an intermediate in the synthesis of more complex organic molecules.
Biology: The compound can be used in studies related to enzyme-catalyzed reactions involving esters.
Medicine: It may serve as a precursor for the synthesis of pharmaceutical compounds.
Industry: The ester is used in the production of fragrances and flavoring agents due to its pleasant aroma.
Comparison with Similar Compounds
Similar Compounds
Ethyl acetate: Another ester with similar reactivity but different applications.
Methyl butyrate: Known for its fruity aroma and used in flavoring agents.
Methyl benzoate: Similar structure but with a different aromatic ring substitution pattern.
Uniqueness
Desmethyl Ketoprofen Methyl Ester is unique due to its specific substitution pattern on the aromatic ring, which imparts distinct chemical and physical properties. This uniqueness makes it valuable in specialized applications where other esters may not be suitable.
Biological Activity
Desmethyl Ketoprofen Methyl Ester (DMKME) is a derivative of ketoprofen, a well-known non-steroidal anti-inflammatory drug (NSAID). This article explores the biological activity of DMKME, focusing on its pharmacological properties, synthesis, and potential therapeutic applications based on recent research findings.
1. Synthesis and Characterization
The synthesis of DMKME typically involves the esterification of desmethyl ketoprofen with methanol. Characterization techniques such as ^1H NMR and infrared (IR) spectroscopy confirm the successful formation of the ester. These techniques reveal characteristic signals corresponding to methoxy protons and aromatic protons, indicating the presence of the desired functional groups .
2.1 Anti-inflammatory Activity
DMKME exhibits significant anti-inflammatory properties. In vitro studies demonstrate its ability to stabilize lysosomal membranes, which is crucial in preventing the release of lysosomal enzymes that contribute to inflammation. This stabilization is associated with a reduction in inflammatory markers in various models .
2.2 Analgesic Effects
In vivo studies using acetic acid-induced writhing tests and formalin-induced paw licking tests show that DMKME effectively alleviates pain. The analgesic effect is comparable to that of ketoprofen but with reduced hepatotoxicity, making it a safer alternative for long-term use in chronic pain management .
3. Toxicity Profile
One of the most notable findings regarding DMKME is its significantly lower hepatotoxicity compared to ketoprofen. In studies assessing organ toxicity, DMKME demonstrated no significant adverse effects on liver function, while traditional ketoprofen showed higher hepatotoxicity levels . This profile suggests that DMKME could be a safer option for patients requiring NSAID therapy.
4. Pharmacokinetics
Research indicates that DMKME has enhanced oral bioavailability compared to its parent compound. In silico analyses predict that DMKME has a higher absorption rate and lower toxicity, making it an ideal candidate for further development . The absorption enhancement ratio (R) for DMKME was observed to be significantly higher than that of ketoprofen, indicating better intestinal permeability .
5.1 Clinical Implications
Recent studies have highlighted the potential of DMKME in treating chronic inflammatory diseases such as arthritis and other pain-related conditions. Its efficacy combined with a reduced side effect profile makes it a promising candidate for clinical applications .
5.2 Comparative Studies
A comparative analysis between DMKME and other NSAIDs reveals that while many NSAIDs exhibit similar anti-inflammatory effects, DMKME's unique profile allows for better patient compliance due to fewer gastrointestinal and hepatic side effects .
6. Data Summary Table
Property | This compound | Ketoprofen |
---|---|---|
Anti-inflammatory Activity | Significant | Significant |
Analgesic Effect | Effective | Effective |
Hepatotoxicity | None | Present |
Oral Bioavailability | Enhanced | Standard |
Absorption Enhancement Ratio | Higher (1.57-fold) | Baseline |
7. Conclusion
This compound represents a significant advancement in the development of safer NSAIDs with effective anti-inflammatory and analgesic properties. Its reduced hepatotoxicity makes it an attractive alternative for patients requiring long-term treatment for chronic pain conditions.
The ongoing research into DMKME's pharmacological profile promises further insights into its therapeutic potential, paving the way for future clinical applications.
Properties
IUPAC Name |
methyl 2-(3-benzoylphenyl)acetate | |
---|---|---|
Source | PubChem | |
URL | https://pubchem.ncbi.nlm.nih.gov | |
Description | Data deposited in or computed by PubChem | |
InChI |
InChI=1S/C16H14O3/c1-19-15(17)11-12-6-5-9-14(10-12)16(18)13-7-3-2-4-8-13/h2-10H,11H2,1H3 | |
Source | PubChem | |
URL | https://pubchem.ncbi.nlm.nih.gov | |
Description | Data deposited in or computed by PubChem | |
InChI Key |
QTXILVCSTXQKLF-UHFFFAOYSA-N | |
Source | PubChem | |
URL | https://pubchem.ncbi.nlm.nih.gov | |
Description | Data deposited in or computed by PubChem | |
Canonical SMILES |
COC(=O)CC1=CC(=CC=C1)C(=O)C2=CC=CC=C2 | |
Source | PubChem | |
URL | https://pubchem.ncbi.nlm.nih.gov | |
Description | Data deposited in or computed by PubChem | |
Molecular Formula |
C16H14O3 | |
Source | PubChem | |
URL | https://pubchem.ncbi.nlm.nih.gov | |
Description | Data deposited in or computed by PubChem | |
DSSTOX Substance ID |
DTXSID70442832 | |
Record name | Methyl (3-benzoylphenyl)acetate | |
Source | EPA DSSTox | |
URL | https://comptox.epa.gov/dashboard/DTXSID70442832 | |
Description | DSSTox provides a high quality public chemistry resource for supporting improved predictive toxicology. | |
Molecular Weight |
254.28 g/mol | |
Source | PubChem | |
URL | https://pubchem.ncbi.nlm.nih.gov | |
Description | Data deposited in or computed by PubChem | |
CAS No. |
24021-44-1 | |
Record name | Methyl (3-benzoylphenyl)acetate | |
Source | EPA DSSTox | |
URL | https://comptox.epa.gov/dashboard/DTXSID70442832 | |
Description | DSSTox provides a high quality public chemistry resource for supporting improved predictive toxicology. | |
Synthesis routes and methods
Procedure details
Retrosynthesis Analysis
AI-Powered Synthesis Planning: Our tool employs the Template_relevance Pistachio, Template_relevance Bkms_metabolic, Template_relevance Pistachio_ringbreaker, Template_relevance Reaxys, Template_relevance Reaxys_biocatalysis model, leveraging a vast database of chemical reactions to predict feasible synthetic routes.
One-Step Synthesis Focus: Specifically designed for one-step synthesis, it provides concise and direct routes for your target compounds, streamlining the synthesis process.
Accurate Predictions: Utilizing the extensive PISTACHIO, BKMS_METABOLIC, PISTACHIO_RINGBREAKER, REAXYS, REAXYS_BIOCATALYSIS database, our tool offers high-accuracy predictions, reflecting the latest in chemical research and data.
Strategy Settings
Precursor scoring | Relevance Heuristic |
---|---|
Min. plausibility | 0.01 |
Model | Template_relevance |
Template Set | Pistachio/Bkms_metabolic/Pistachio_ringbreaker/Reaxys/Reaxys_biocatalysis |
Top-N result to add to graph | 6 |
Feasible Synthetic Routes
Disclaimer and Information on In-Vitro Research Products
Please be aware that all articles and product information presented on BenchChem are intended solely for informational purposes. The products available for purchase on BenchChem are specifically designed for in-vitro studies, which are conducted outside of living organisms. In-vitro studies, derived from the Latin term "in glass," involve experiments performed in controlled laboratory settings using cells or tissues. It is important to note that these products are not categorized as medicines or drugs, and they have not received approval from the FDA for the prevention, treatment, or cure of any medical condition, ailment, or disease. We must emphasize that any form of bodily introduction of these products into humans or animals is strictly prohibited by law. It is essential to adhere to these guidelines to ensure compliance with legal and ethical standards in research and experimentation.