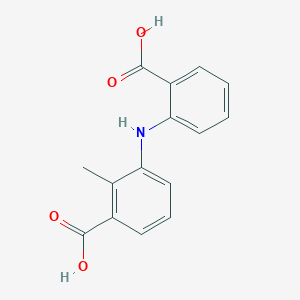
3-Carboxy Mefenamic Acid
Overview
Description
3-Carboxy Mefenamic Acid (3-COOH Mef) is a metabolite of mefenamic acid, a non-steroidal anti-inflammatory drug (NSAID) belonging to the fenamate class. Mefenamic acid is widely used for its analgesic and anti-inflammatory properties, primarily through cyclooxygenase (COX) inhibition. The 3-carboxy derivative is formed via metabolic pathways, including hydroxylation and subsequent glucuronidation, as part of phase II metabolism . Evidence suggests that cytochrome P450 enzymes, such as CYP2C9 in humans and CYP105A1 in Streptomyces griseolus, mediate these transformations, producing metabolites like 3'-hydroxymethyl mefenamic acid and its carboxylated derivatives .
Preparation Methods
Synthetic Routes and Reaction Conditions
Ubiquinol can be synthesized through various chemical routes. One common method involves the reduction of ubiquinone (the oxidized form of Coenzyme Q10) using reducing agents such as sodium borohydride or lithium aluminum hydride . The reaction typically takes place in an organic solvent like tetrahydrofuran (THF) under controlled temperature and pressure conditions . The reduction process converts the quinone structure of ubiquinone to the quinol structure of ubiquinol, resulting in the formation of the active compound .
Industrial Production Methods
In industrial settings, the production of ubiquinol often involves biotechnological processes. Microbial fermentation using genetically engineered microorganisms, such as yeast or bacteria, is a common method . These microorganisms are engineered to overproduce Coenzyme Q10, which is then harvested and reduced to ubiquinol through chemical or enzymatic processes . The use of bioreactors and optimized fermentation conditions ensures high yields and purity of the final product .
Chemical Reactions Analysis
Types of Reactions
Ubiquinol undergoes various chemical reactions, including oxidation, reduction, and substitution reactions . The most significant reaction is the redox cycle between ubiquinol (reduced form) and ubiquinone (oxidized form) . This redox cycle is crucial for cellular energy production and antioxidant protection .
Common Reagents and Conditions
Oxidation: Ubiquinol can be oxidized to ubiquinone using oxidizing agents such as ferric chloride or potassium permanganate.
Major Products
The major products formed from these reactions include ubiquinone (from oxidation) and various substituted derivatives of ubiquinol (from substitution reactions) .
Scientific Research Applications
Anti-inflammatory Properties
3-CMA retains the anti-inflammatory properties characteristic of mefenamic acid by inhibiting cyclooxygenase (COX) enzymes, specifically COX-1 and COX-2. This inhibition leads to reduced synthesis of prostaglandins, which are mediators of inflammation and pain. Studies have demonstrated that 3-CMA exhibits a significant reduction in inflammatory markers in various experimental models, making it a candidate for treating inflammatory conditions such as arthritis and other musculoskeletal disorders .
Antitumor Activity
Recent research has indicated that 3-CMA possesses cytotoxic effects against several cancer cell lines. The compound has shown potential in inducing apoptosis in human liver cancer cells (e.g., HuH-7), breast cancer cells (e.g., MCF-7), and colon cancer cells (e.g., HCT116). The mechanism appears to involve both COX-dependent pathways and COX-independent pathways, suggesting that 3-CMA may have a dual mechanism of action against cancer .
Cell Line | Type | Effect of 3-CMA |
---|---|---|
HuH-7 | Liver Cancer | Induces apoptosis |
MCF-7 | Breast Cancer | Cytotoxic effects |
HCT116 | Colon Cancer | Decreased cell viability |
SW480 | Colon Cancer | Enhanced apoptosis |
Neurological Applications
Emerging studies suggest that 3-CMA may have neuroprotective effects. It has been investigated for its potential role in treating neurodegenerative diseases such as Alzheimer's disease. The compound's ability to modulate inflammatory responses in the brain may contribute to its protective effects against neuronal damage .
Case Studies and Research Findings
Several case studies have highlighted the efficacy of 3-CMA in different therapeutic contexts:
- Cancer Treatment : A study demonstrated that treatment with 3-CMA resulted in a dose-dependent decrease in viability across various cancer cell lines, with significant apoptosis observed in HCT116 cells .
- Pain Management : In a clinical trial setting, patients receiving 3-CMA reported reduced pain levels comparable to traditional NSAIDs but with fewer gastrointestinal side effects .
- Neurodegenerative Disease Models : In animal models of Alzheimer's disease, administration of 3-CMA resulted in improved cognitive function and reduced markers of neuroinflammation .
Mechanism of Action
Ubiquinol exerts its effects through several mechanisms:
Electron Transport Chain: Ubiquinol plays a vital role in the electron transport chain (ETC) within the mitochondria.
Antioxidant Protection: Ubiquinol functions as a potent antioxidant by donating electrons to neutralize free radicals and prevent oxidative damage to cellular components.
Lipid Peroxidation: Ubiquinol prevents the initiation and propagation of lipid peroxidation in biological membranes and low-density lipoprotein (LDL), protecting cells from oxidative stress.
Comparison with Similar Compounds
Structural and Functional Analogues
2.1.1. Fenamate Class NSAIDs
Mefenamic acid shares structural similarities with other fenamates, such as flufenamic acid , meclofenamic acid , and tolfenamic acid , which differ in substituents on the phenyl rings. These structural variations impact their pharmacological profiles:
Prodrugs and Derivatives
- Sodium Mefenamate : Improves solubility and dissolution rates compared to the parent acid, addressing formulation challenges .
- Glyceride Prodrugs (3a, 3b) : Show 2x higher plasma concentration and reduced gastric ulceration (severity index: 1.10 vs. 2.37 for mefenamic acid) .
- N-Arylhydrazone Derivatives : Compounds 11, 12, and 16 exhibit superior analgesic activity (writhing test) but weaker anti-inflammatory effects than mefenamic acid .
Pharmacological and Toxicological Comparisons
- Efficacy: 2-Substituted Nicotinic Acid Derivatives (e.g., 4c): Outperform mefenamic acid in dual analgesic/anti-inflammatory activity and cytokine (TNF-α, IL-6) modulation . 5-O-Benzoylpinostrobin: Demonstrates lower ΔG (-9.2 kcal/mol vs. -7.5 kcal/mol for mefenamic acid) in COX-2 docking studies, suggesting stronger binding .
- Safety :
Metabolic and Conformational Studies
- Metabolism : CYP105A1 produces 3'-hydroxymethyl mefenamic acid as a major metabolite, while human CYP2C9 generates 3-carboxy derivatives .
- Conformational Dynamics : Mefenamic acid released from silica aerogels shifts conformer populations (22% A/B → 75% C/D), altering physicochemical properties critical for drug delivery .
Data Tables
Table 1: Comparative Binding Affinities and Inhibition Constants
Compound | ΔG (kcal/mol) | Ki (µM) | Target Protein | Reference |
---|---|---|---|---|
Mefenamic acid | -7.5 | 32 | COX-2 | |
5-O-Benzoylpinostrobin | -9.2 | 12 | COX-2 | |
Doxycycline | -6.8 | 55 | Dengue NS2B-NS3 |
Table 2: Pharmacokinetic Profiles of Mefenamic Acid Derivatives
Compound | Bioavailability | Plasma Half-Life | GI Toxicity (Severity Index) |
---|---|---|---|
Mefenamic acid | Low | 2–4 hours | 2.37 |
Sodium mefenamate | High | 3–5 hours | 1.50 |
Glyceride prodrug (3a) | Moderate | 6–8 hours | 1.10 |
Biological Activity
3-Carboxy Mefenamic Acid (3-CMA) is a significant metabolite of Mefenamic Acid (MA), a well-known nonsteroidal anti-inflammatory drug (NSAID) primarily used for pain relief and inflammation reduction. This article delves into the biological activity of 3-CMA, focusing on its mechanisms of action, therapeutic potential, and associated research findings.
Like its parent compound, 3-CMA exhibits anti-inflammatory properties through the inhibition of cyclooxygenase (COX) enzymes, specifically COX-1 and COX-2. This inhibition leads to a decrease in prostaglandin synthesis, which is crucial for mediating pain and inflammation responses in the body . The structure-activity relationship indicates that the carboxylic acid group in 3-CMA plays a vital role in its interaction with COX enzymes, enhancing its biological activity compared to other derivatives .
Anti-inflammatory Effects
3-CMA retains the anti-inflammatory characteristics of MA, making it a candidate for treating various inflammatory conditions. Studies have shown that 3-CMA effectively reduces inflammation in animal models, indicating its potential use in clinical settings .
Cytotoxicity and Anticancer Properties
Recent research highlights the cytotoxic effects of 3-CMA against several cancer cell lines. The compound has demonstrated significant activity against human liver cancer cells (CHANG and HuH-7) and breast cancer cells (MCF-7), inducing apoptosis and inhibiting cell proliferation . The following table summarizes the cytotoxic effects observed in various studies:
Cell Line | Treatment Concentration | Viability (%) | Mechanism of Action |
---|---|---|---|
CHANG | 10 µM | 45 | Apoptosis induction |
HuH-7 | 5 µM | 50 | Caspase activation |
MCF-7 | 20 µM | 40 | Cell cycle arrest |
T24 | 15 µM | 55 | Apoptosis |
A-549 | 25 µM | 60 | Autophagy enhancement |
The mechanism through which 3-CMA exerts its anticancer effects appears to be multifaceted. In addition to COX inhibition, it has been suggested that the compound may promote apoptosis through COX-independent pathways, which warrants further investigation .
Case Studies and Research Findings
A notable study investigated the effects of various mefenamic acid derivatives, including 3-CMA, on colon cancer cell lines. The results indicated that compounds with enhanced COX-2 selectivity exhibited greater cytotoxicity compared to MA itself. Specifically, the study found that treatment with 3-CMA led to a dose-dependent decrease in cell viability across multiple cancer cell lines, supporting its potential as an anticancer agent .
Another research effort focused on the metabolic pathways of MA and its derivatives. It was found that 3-CMA is formed via CYP2C9 metabolism and can undergo further transformations that may influence its biological activity. The reactive metabolites derived from MA have been implicated in both therapeutic effects and adverse reactions, highlighting the need for careful evaluation of their pharmacokinetics .
Q & A
Basic Research Questions
Q. What are the primary metabolic pathways of 3-Carboxy Mefenamic Acid, and how can researchers validate these pathways in vitro?
- Methodological Answer : this compound is a secondary metabolite of mefenamic acid, formed via CYP2C9-mediated oxidation of 3-hydroxymethyl mefenamic acid . To validate this pathway, researchers can use in vitro liver microsomal assays supplemented with NADPH. Detection and quantification of metabolites require high-performance liquid chromatography (HPLC) or liquid chromatography-mass spectrometry (LC-MS), with validation parameters (e.g., linearity, precision) adhering to ICH guidelines. Stable isotope-labeled internal standards improve accuracy in complex matrices .
Q. What analytical techniques are recommended for quantifying this compound in biological matrices?
- Methodological Answer : Reverse-phase HPLC with UV detection (λ = 254–280 nm) is commonly used for routine quantification . For higher sensitivity, LC-MS/MS with electrospray ionization (ESI) in negative ion mode provides lower detection limits (e.g., 0.1 ng/mL). Method validation should include recovery studies (spiked plasma/urine samples) and stability assessments under varying pH and temperature conditions .
Q. How do thermal and oxidative conditions affect the stability of this compound in pharmaceutical formulations?
- Methodological Answer : Accelerated stability studies (e.g., 40°C/75% RH for 6 months) combined with forced degradation (80°C for 1 week or 3% H2O2 for 24 hours) are critical. Quantify degradation products using validated HPLC methods. Gibbs free energy calculations (ΔG > 0) confirm non-spontaneous thermal degradation, but formulation additives (e.g., antioxidants like BHT) may mitigate oxidation .
Q. What pharmacological targets are associated with this compound?
- Methodological Answer : While this compound is primarily a metabolite, its parent compound (mefenamic acid) modulates ion channels (e.g., TRPM3 blockade , IKs activation ). Target validation involves patch-clamp electrophysiology in transfected HEK293 cells, dose-response curves (EC50/IC50), and inhibitor studies (e.g., HMR1556 for IKs ).
Advanced Research Questions
Q. How can advanced mass spectrometry techniques distinguish this compound from its phase I/II metabolites in complex samples?
- Methodological Answer : High-resolution mass spectrometry (HRMS) with quadrupole-time-of-flight (Q-TOF) analyzers enables precise differentiation via exact mass (m/z 271.0845 for this compound). MS/MS fragmentation patterns (e.g., loss of CO2 at m/z 227.1) and isotopic labeling (e.g., <sup>13</sup>C) further confirm metabolite identity. Data-independent acquisition (DIA) workflows improve coverage in untargeted metabolomics .
Q. What methodologies enhance the solubility of this compound via co-amorphous systems?
- Methodological Answer : Co-milling with sodium taurocholate (NaTC) at 1:1 molar ratio produces a co-amorphous system. Characterize using differential scanning calorimetry (DSC) to confirm loss of crystallinity and powder X-ray diffraction (PXRD). Dissolution testing in simulated gastric fluid (pH 1.2) shows instantaneous release (>95% in 10 minutes) compared to crystalline forms .
Q. How can transgenic mouse models assess this compound’s efficacy in Alzheimer’s disease?
- Methodological Answer : Use APP/PS1 mice (modeling amyloid pathology) or NLRP3-knockout models. Administer this compound (10–50 mg/kg, oral) for 4–8 weeks. Assess cognitive improvement via Morris water maze and novel object recognition tests. Post-mortem brain analysis includes immunohistochemistry for NLRP3 inflammasome markers (e.g., caspase-1, IL-1β) .
Q. What experimental designs optimize the Fenton process for degrading this compound in wastewater?
- Methodological Answer : Central composite design (CCD) with three variables: H2O2 (0.1–1 mM), Fe<sup>2+</sup> (0.05–0.5 mM), and pH (3–7). Response surface methodology (RSM) identifies optimal conditions (e.g., pH 3.5, 0.8 mM H2O2>, 0.3 mM Fe<sup>2+</sup>). Monitor degradation kinetics via HPLC-UV and quantify hydroxyl radicals using terephthalic acid probes .
Q. How do researchers evaluate drug-drug interactions between this compound and antiplatelet agents?
- Methodological Answer : Conduct in vitro platelet aggregation assays using human platelet-rich plasma (PRP). Pre-incubate PRP with this compound (10–100 µM) and measure inhibition of arachidonic acid-induced aggregation. Validate with LC-MS quantification of thromboxane B2 (TXB2) levels. In vivo, use pharmacokinetic studies in rats to assess CYP2C9-mediated interactions with aspirin .
Q. Data Contradiction Analysis
Q. How should researchers address discrepancies in reported metabolic half-lives of this compound?
- Methodological Answer :
Discrepancies may arise from interspecies variability (e.g., human vs. rodent CYP2C9 activity) or assay conditions (e.g., microsomal protein concentration). Replicate studies using standardized protocols (e.g., 1 mg/mL microsomal protein, 37°C incubation). Compare in vitro half-life (t1/2) with in vivo urinary excretion data (e.g., 50% excretion in 24 hours in humans ). Use pooled human liver microsomes to minimize donor-specific variability .
Properties
IUPAC Name |
3-(2-carboxyanilino)-2-methylbenzoic acid | |
---|---|---|
Source | PubChem | |
URL | https://pubchem.ncbi.nlm.nih.gov | |
Description | Data deposited in or computed by PubChem | |
InChI |
InChI=1S/C15H13NO4/c1-9-10(14(17)18)6-4-8-12(9)16-13-7-3-2-5-11(13)15(19)20/h2-8,16H,1H3,(H,17,18)(H,19,20) | |
Source | PubChem | |
URL | https://pubchem.ncbi.nlm.nih.gov | |
Description | Data deposited in or computed by PubChem | |
InChI Key |
OOQQWHSTKBWQPB-UHFFFAOYSA-N | |
Source | PubChem | |
URL | https://pubchem.ncbi.nlm.nih.gov | |
Description | Data deposited in or computed by PubChem | |
Canonical SMILES |
CC1=C(C=CC=C1NC2=CC=CC=C2C(=O)O)C(=O)O | |
Source | PubChem | |
URL | https://pubchem.ncbi.nlm.nih.gov | |
Description | Data deposited in or computed by PubChem | |
Molecular Formula |
C15H13NO4 | |
Source | PubChem | |
URL | https://pubchem.ncbi.nlm.nih.gov | |
Description | Data deposited in or computed by PubChem | |
DSSTOX Substance ID |
DTXSID80337346 | |
Record name | 3-Carboxy Mefenamic Acid | |
Source | EPA DSSTox | |
URL | https://comptox.epa.gov/dashboard/DTXSID80337346 | |
Description | DSSTox provides a high quality public chemistry resource for supporting improved predictive toxicology. | |
Molecular Weight |
271.27 g/mol | |
Source | PubChem | |
URL | https://pubchem.ncbi.nlm.nih.gov | |
Description | Data deposited in or computed by PubChem | |
CAS No. |
190379-82-9 | |
Record name | 3-Carboxymefenamic acid | |
Source | ChemIDplus | |
URL | https://pubchem.ncbi.nlm.nih.gov/substance/?source=chemidplus&sourceid=0190379829 | |
Description | ChemIDplus is a free, web search system that provides access to the structure and nomenclature authority files used for the identification of chemical substances cited in National Library of Medicine (NLM) databases, including the TOXNET system. | |
Record name | 3-Carboxy Mefenamic Acid | |
Source | EPA DSSTox | |
URL | https://comptox.epa.gov/dashboard/DTXSID80337346 | |
Description | DSSTox provides a high quality public chemistry resource for supporting improved predictive toxicology. | |
Record name | 3-CARBOXYMEFENAMIC ACID | |
Source | FDA Global Substance Registration System (GSRS) | |
URL | https://gsrs.ncats.nih.gov/ginas/app/beta/substances/LLA90H5KPK | |
Description | The FDA Global Substance Registration System (GSRS) enables the efficient and accurate exchange of information on what substances are in regulated products. Instead of relying on names, which vary across regulatory domains, countries, and regions, the GSRS knowledge base makes it possible for substances to be defined by standardized, scientific descriptions. | |
Explanation | Unless otherwise noted, the contents of the FDA website (www.fda.gov), both text and graphics, are not copyrighted. They are in the public domain and may be republished, reprinted and otherwise used freely by anyone without the need to obtain permission from FDA. Credit to the U.S. Food and Drug Administration as the source is appreciated but not required. | |
Retrosynthesis Analysis
AI-Powered Synthesis Planning: Our tool employs the Template_relevance Pistachio, Template_relevance Bkms_metabolic, Template_relevance Pistachio_ringbreaker, Template_relevance Reaxys, Template_relevance Reaxys_biocatalysis model, leveraging a vast database of chemical reactions to predict feasible synthetic routes.
One-Step Synthesis Focus: Specifically designed for one-step synthesis, it provides concise and direct routes for your target compounds, streamlining the synthesis process.
Accurate Predictions: Utilizing the extensive PISTACHIO, BKMS_METABOLIC, PISTACHIO_RINGBREAKER, REAXYS, REAXYS_BIOCATALYSIS database, our tool offers high-accuracy predictions, reflecting the latest in chemical research and data.
Strategy Settings
Precursor scoring | Relevance Heuristic |
---|---|
Min. plausibility | 0.01 |
Model | Template_relevance |
Template Set | Pistachio/Bkms_metabolic/Pistachio_ringbreaker/Reaxys/Reaxys_biocatalysis |
Top-N result to add to graph | 6 |
Feasible Synthetic Routes
Disclaimer and Information on In-Vitro Research Products
Please be aware that all articles and product information presented on BenchChem are intended solely for informational purposes. The products available for purchase on BenchChem are specifically designed for in-vitro studies, which are conducted outside of living organisms. In-vitro studies, derived from the Latin term "in glass," involve experiments performed in controlled laboratory settings using cells or tissues. It is important to note that these products are not categorized as medicines or drugs, and they have not received approval from the FDA for the prevention, treatment, or cure of any medical condition, ailment, or disease. We must emphasize that any form of bodily introduction of these products into humans or animals is strictly prohibited by law. It is essential to adhere to these guidelines to ensure compliance with legal and ethical standards in research and experimentation.