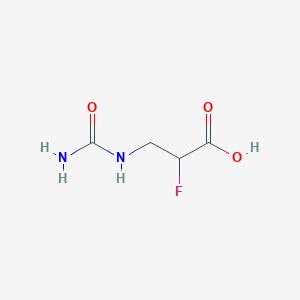
alpha-Fluoro-beta-ureidopropionic acid
Overview
Description
Barnidipine (hydrochloride) is a long-acting calcium channel blocker belonging to the dihydropyridine group. It is primarily used in the treatment of hypertension. Barnidipine exhibits high affinity for calcium channels in the smooth muscle cells of the vascular wall, leading to vasodilation and reduced blood pressure .
Scientific Research Applications
Barnidipine (hydrochloride) has several scientific research applications:
Chemistry: Used as a model compound in the study of calcium channel blockers.
Biology: Investigated for its effects on cellular calcium channels and vascular smooth muscle cells.
Medicine: Used in clinical trials for the treatment of hypertension and related cardiovascular conditions.
Industry: Employed in the development of new antihypertensive drugs and formulations
Mechanism of Action
Target of Action
The primary target of alpha-Fluoro-beta-ureidopropionic acid is the enzyme Beta-ureidopropionase (UPB1) . This enzyme plays a crucial role in the pyrimidine degradation pathway, where it converts N-carbamyl-beta-aminoisobutyric acid and N-carbamyl-beta-alanine to beta-aminoisobutyric acid and beta-alanine, respectively .
Mode of Action
This compound interacts with its target, Beta-ureidopropionase, and is converted into alpha-Fluoro-beta-alanine , along with the release of carbon dioxide and ammonia . This reaction is part of the enzyme’s normal function in the pyrimidine degradation pathway .
Biochemical Pathways
The action of this compound affects the pyrimidine degradation pathway . This pathway is responsible for the breakdown of pyrimidine bases, which are key components of nucleic acids. The conversion of this compound by Beta-ureidopropionase contributes to the production of beta-alanine, a component of several biochemically significant compounds .
Pharmacokinetics
The pharmacokinetics of this compound are closely related to those of 5-fluorouracil (5-FU), a widely used anticancer drug . The compound is metabolized in the body, leading to the production of various metabolites, including alpha-Fluoro-beta-alanine . The systemic exposure to these metabolites can influence the drug’s bioavailability and efficacy .
Result of Action
The conversion of this compound to alpha-Fluoro-beta-alanine is a key step in the metabolism of the drug 5-FU . This process can influence the therapeutic and adverse effects of 5-FU, as the metabolites produced can contribute to the drug’s anticancer activity or cause toxicity .
Action Environment
The action of this compound can be influenced by various environmental factors. For instance, the activity of Beta-ureidopropionase, the enzyme that metabolizes the compound, can be affected by factors such as pH and temperature . Additionally, the presence of other substances in the body, such as other drugs or endogenous compounds, can also influence the compound’s action, efficacy, and stability .
Safety and Hazards
Future Directions
Biochemical Analysis
Biochemical Properties
Alpha-Fluoro-beta-ureidopropionic acid interacts with several enzymes and proteins. One such enzyme is Beta-ureidopropionase, which converts N-carbamyl-beta-aminoisobutyric acid and N-carbamyl-beta-alanine to beta-aminoisobutyric acid and beta-alanine, respectively . The interactions between this compound and these biomolecules are crucial for its role in biochemical reactions .
Cellular Effects
This compound influences various types of cells and cellular processes. It impacts cell function, including cell signaling pathways, gene expression, and cellular metabolism
Molecular Mechanism
At the molecular level, this compound exerts its effects through binding interactions with biomolecules, enzyme inhibition or activation, and changes in gene expression . The exact mechanism of action of this compound is complex and involves multiple biochemical pathways .
Temporal Effects in Laboratory Settings
In laboratory settings, the effects of this compound change over time. This includes information on the product’s stability, degradation, and any long-term effects on cellular function observed in in vitro or in vivo studies . More detailed studies are needed to fully understand these temporal effects.
Dosage Effects in Animal Models
The effects of this compound vary with different dosages in animal models . These effects could include any threshold effects observed in these studies, as well as any toxic or adverse effects at high doses
Metabolic Pathways
This compound is involved in several metabolic pathways. It interacts with enzymes such as Beta-ureidopropionase and affects metabolic flux or metabolite levels
Transport and Distribution
This compound is transported and distributed within cells and tissues . It interacts with various transporters or binding proteins, and these interactions can affect its localization or accumulation
Subcellular Localization
It could involve targeting signals or post-translational modifications that direct it to specific compartments or organelles
Preparation Methods
Synthetic Routes and Reaction Conditions: The synthesis of barnidipine (hydrochloride) involves several steps:
Reaction of 3-hydroxypropionitrile with ketene dimer: to obtain an intermediate compound.
Reaction of the intermediate with m-nitrobenzaldehyde: to form another intermediate.
Reaction with beta-amino ethyl crotonate: to produce a further intermediate.
Hydrolysis by an alkali: to obtain a compound.
Resolution with a chiral organic base: to isolate the desired enantiomer.
Reaction with benzyl pyrrole alcohol: to form the final compound.
Addition of the final compound to a hydrochloric acid solution: to obtain barnidipine (hydrochloride).
Industrial Production Methods: The industrial production of barnidipine (hydrochloride) follows the same synthetic route but is optimized for higher yields and purity. The process involves mild reaction conditions, easily separable and purifiable products, and the use of readily available raw materials .
Chemical Reactions Analysis
Types of Reactions: Barnidipine (hydrochloride) undergoes various chemical reactions, including:
Oxidation: Involves the addition of oxygen or removal of hydrogen.
Reduction: Involves the addition of hydrogen or removal of oxygen.
Substitution: Involves the replacement of one functional group with another.
Common Reagents and Conditions:
Oxidation: Common reagents include potassium permanganate and hydrogen peroxide.
Reduction: Common reagents include lithium aluminum hydride and sodium borohydride.
Substitution: Common reagents include halogens and nucleophiles.
Major Products: The major products formed from these reactions depend on the specific reagents and conditions used. For example, oxidation may yield carboxylic acids, while reduction may yield alcohols .
Comparison with Similar Compounds
Barnidipine (hydrochloride) is compared with other calcium channel blockers such as amlodipine, nitrendipine, atenolol, enalapril, and hydrochlorothiazide. Unlike these compounds, barnidipine consists of a single enantiomer, offering higher pharmacological selectivity and fewer side effects . Similar compounds include:
- Amlodipine
- Nitrendipine
- Atenolol
- Enalapril
- Hydrochlorothiazide
Barnidipine’s unique single enantiomer composition provides it with a distinct advantage in terms of efficacy and safety .
Properties
IUPAC Name |
3-(carbamoylamino)-2-fluoropropanoic acid | |
---|---|---|
Source | PubChem | |
URL | https://pubchem.ncbi.nlm.nih.gov | |
Description | Data deposited in or computed by PubChem | |
InChI |
InChI=1S/C4H7FN2O3/c5-2(3(8)9)1-7-4(6)10/h2H,1H2,(H,8,9)(H3,6,7,10) | |
Source | PubChem | |
URL | https://pubchem.ncbi.nlm.nih.gov | |
Description | Data deposited in or computed by PubChem | |
InChI Key |
FKTHAKABFGARQH-UHFFFAOYSA-N | |
Source | PubChem | |
URL | https://pubchem.ncbi.nlm.nih.gov | |
Description | Data deposited in or computed by PubChem | |
Canonical SMILES |
C(C(C(=O)O)F)NC(=O)N | |
Source | PubChem | |
URL | https://pubchem.ncbi.nlm.nih.gov | |
Description | Data deposited in or computed by PubChem | |
Molecular Formula |
C4H7FN2O3 | |
Source | PubChem | |
URL | https://pubchem.ncbi.nlm.nih.gov | |
Description | Data deposited in or computed by PubChem | |
DSSTOX Substance ID |
DTXSID50964507 | |
Record name | 2-Fluoro-3-{[hydroxy(imino)methyl]amino}propanoic acid | |
Source | EPA DSSTox | |
URL | https://comptox.epa.gov/dashboard/DTXSID50964507 | |
Description | DSSTox provides a high quality public chemistry resource for supporting improved predictive toxicology. | |
Molecular Weight |
150.11 g/mol | |
Source | PubChem | |
URL | https://pubchem.ncbi.nlm.nih.gov | |
Description | Data deposited in or computed by PubChem | |
CAS No. |
5006-64-4 | |
Record name | α-Fluoro-β-ureidopropionic acid | |
Source | CAS Common Chemistry | |
URL | https://commonchemistry.cas.org/detail?cas_rn=5006-64-4 | |
Description | CAS Common Chemistry is an open community resource for accessing chemical information. Nearly 500,000 chemical substances from CAS REGISTRY cover areas of community interest, including common and frequently regulated chemicals, and those relevant to high school and undergraduate chemistry classes. This chemical information, curated by our expert scientists, is provided in alignment with our mission as a division of the American Chemical Society. | |
Explanation | The data from CAS Common Chemistry is provided under a CC-BY-NC 4.0 license, unless otherwise stated. | |
Record name | alpha-Fluoro-beta-ureidopropionic acid | |
Source | ChemIDplus | |
URL | https://pubchem.ncbi.nlm.nih.gov/substance/?source=chemidplus&sourceid=0005006644 | |
Description | ChemIDplus is a free, web search system that provides access to the structure and nomenclature authority files used for the identification of chemical substances cited in National Library of Medicine (NLM) databases, including the TOXNET system. | |
Record name | 2-Fluoro-3-{[hydroxy(imino)methyl]amino}propanoic acid | |
Source | EPA DSSTox | |
URL | https://comptox.epa.gov/dashboard/DTXSID50964507 | |
Description | DSSTox provides a high quality public chemistry resource for supporting improved predictive toxicology. | |
Record name | 3-[(Aminocarbonyl)amino]-2-fluoro-propanoic Acid | |
Source | European Chemicals Agency (ECHA) | |
URL | https://echa.europa.eu/information-on-chemicals | |
Description | The European Chemicals Agency (ECHA) is an agency of the European Union which is the driving force among regulatory authorities in implementing the EU's groundbreaking chemicals legislation for the benefit of human health and the environment as well as for innovation and competitiveness. | |
Explanation | Use of the information, documents and data from the ECHA website is subject to the terms and conditions of this Legal Notice, and subject to other binding limitations provided for under applicable law, the information, documents and data made available on the ECHA website may be reproduced, distributed and/or used, totally or in part, for non-commercial purposes provided that ECHA is acknowledged as the source: "Source: European Chemicals Agency, http://echa.europa.eu/". Such acknowledgement must be included in each copy of the material. ECHA permits and encourages organisations and individuals to create links to the ECHA website under the following cumulative conditions: Links can only be made to webpages that provide a link to the Legal Notice page. | |
Record name | .ALPHA.-FLUOROURIDOPROPIONIC ACID | |
Source | FDA Global Substance Registration System (GSRS) | |
URL | https://gsrs.ncats.nih.gov/ginas/app/beta/substances/0BXG1I9G0W | |
Description | The FDA Global Substance Registration System (GSRS) enables the efficient and accurate exchange of information on what substances are in regulated products. Instead of relying on names, which vary across regulatory domains, countries, and regions, the GSRS knowledge base makes it possible for substances to be defined by standardized, scientific descriptions. | |
Explanation | Unless otherwise noted, the contents of the FDA website (www.fda.gov), both text and graphics, are not copyrighted. They are in the public domain and may be republished, reprinted and otherwise used freely by anyone without the need to obtain permission from FDA. Credit to the U.S. Food and Drug Administration as the source is appreciated but not required. | |
Retrosynthesis Analysis
AI-Powered Synthesis Planning: Our tool employs the Template_relevance Pistachio, Template_relevance Bkms_metabolic, Template_relevance Pistachio_ringbreaker, Template_relevance Reaxys, Template_relevance Reaxys_biocatalysis model, leveraging a vast database of chemical reactions to predict feasible synthetic routes.
One-Step Synthesis Focus: Specifically designed for one-step synthesis, it provides concise and direct routes for your target compounds, streamlining the synthesis process.
Accurate Predictions: Utilizing the extensive PISTACHIO, BKMS_METABOLIC, PISTACHIO_RINGBREAKER, REAXYS, REAXYS_BIOCATALYSIS database, our tool offers high-accuracy predictions, reflecting the latest in chemical research and data.
Strategy Settings
Precursor scoring | Relevance Heuristic |
---|---|
Min. plausibility | 0.01 |
Model | Template_relevance |
Template Set | Pistachio/Bkms_metabolic/Pistachio_ringbreaker/Reaxys/Reaxys_biocatalysis |
Top-N result to add to graph | 6 |
Feasible Synthetic Routes
Q1: How is alpha-Fluoro-beta-ureidopropionic acid formed in the body?
A: this compound is formed as a metabolite during the breakdown of 5-fluorouracil (5-FU). Following the administration of 5-FU, it is rapidly metabolized, with a significant portion undergoing catabolism. The enzyme dihydropyrimidine dehydrogenase (DPD) catalyzes the first step in this catabolic pathway, converting 5-FU into dihydrofluorouracil (FUH2). FUH2 is further metabolized into this compound (FUPA) [, ].
Q2: What is the significance of this compound formation in 5-FU therapy?
A: The formation of FUPA signifies the breakdown and inactivation of 5-FU in the body. This catabolic pathway significantly reduces the amount of 5-FU available to exert its antitumor effects [, ]. Therefore, strategies to modulate 5-FU catabolism, such as using DPD inhibitors like 5-ethynyluracil (EU), are being investigated to enhance the therapeutic efficacy of 5-FU [, ].
Q3: Can this compound itself be detected, and if so, how?
A: Yes, this compound can be directly detected and quantified using advanced analytical techniques like high-performance liquid chromatography (HPLC) and 19F nuclear magnetic resonance (NMR) spectroscopy [, , ]. These techniques have been instrumental in characterizing the metabolic profile of 5-FU in various biological samples, including plasma, urine, and tissue extracts.
Q4: How do researchers study the impact of this compound formation in preclinical models?
A: Researchers use in vivo models like mice bearing colon 38 tumors to investigate the effects of modulating this compound formation on 5-FU metabolism []. By administering compounds like EU before 5-FU, they can observe changes in the levels of this compound and other metabolites in different tissues using 19F NMR spectroscopy. These studies provide valuable insights into how altering 5-FU catabolism can impact its availability and potential antitumor activity.
Q5: What happens to this compound after it is formed?
A: this compound is further metabolized into alpha-fluoro-beta-alanine (FBAL) [, ]. FBAL is then excreted in the urine, representing a significant route of elimination for the breakdown products of 5-FU.
Q6: Does the formation of this compound vary across species?
A: Yes, the metabolic profile of 5-FU, including the formation and excretion of this compound, can vary significantly across species. For example, studies using 19F NMR spectroscopy have shown distinct differences in the urinary excretion patterns of FUPA and its metabolites between rats, mice, and humans [].
Q7: Are there other drugs or compounds that influence the metabolism of 5-FU and the formation of this compound ?
A: Yes, several compounds can influence 5-FU metabolism. For instance, using modulators like methotrexate, alpha-interferon, or high-dose leucovorin alongside 5-FU led to an increased ratio of anabolites to catabolites in murine colon carcinoma models []. This suggests a potential shift in 5-FU metabolism towards pathways favoring its antitumor activity. Conversely, N-(phosphonacetyl)-L-aspartate decreased the anabolite:catabolite ratio, potentially reducing 5-FU efficacy [].
Disclaimer and Information on In-Vitro Research Products
Please be aware that all articles and product information presented on BenchChem are intended solely for informational purposes. The products available for purchase on BenchChem are specifically designed for in-vitro studies, which are conducted outside of living organisms. In-vitro studies, derived from the Latin term "in glass," involve experiments performed in controlled laboratory settings using cells or tissues. It is important to note that these products are not categorized as medicines or drugs, and they have not received approval from the FDA for the prevention, treatment, or cure of any medical condition, ailment, or disease. We must emphasize that any form of bodily introduction of these products into humans or animals is strictly prohibited by law. It is essential to adhere to these guidelines to ensure compliance with legal and ethical standards in research and experimentation.